Internal Standard Considerations for Protein Therapeutic Bioanalytical LC-MS/MS Assays
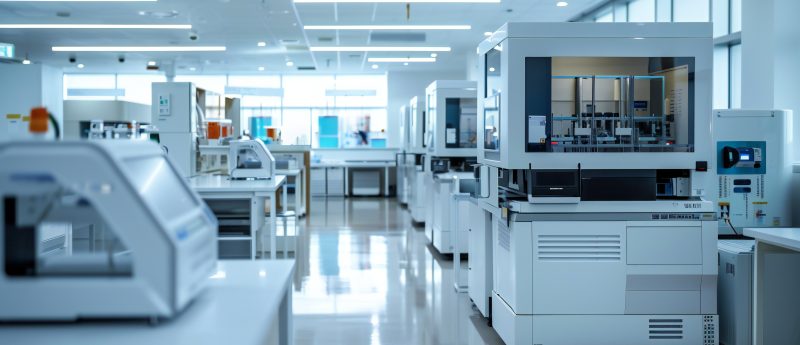
William R Mylott, Jr. is the Associate Director of the Biologics by LC–MS group at PPD Inc. located in Richmond, VA, USA. William received his B.Sc. in Chemistry from the State University of New York (USA), College at Brockport in 1992. William has over 24 years of experience in the areas of analytical and bioanalytical chemistry involving traditional small-molecule and large-molecule (biologics) therapeutics. In his current role, he is responsible for the development and validation of bioanalytical methods for both small and biologic-based therapeutics which include peptides, monoclonal antibodies (mAb), antibody–drug conjugates and other protein/peptide constructs.
The development of protein-based therapeutics has skyrocketed over the past two decades. According to a report by BCC Research, global sales of biologics topped $200 billion in 2013 and are expected to exceed $386 billion by the end of 2019 [1]. As such, there is increasing demand for the development and validation of accurate, precise and robust bioanalytical methods to support pharmacokinetic and toxicokinetic studies and that are capable of withstanding regulatory review. Traditionally, ligand-binding assays have been the method of choice for the quantitation of biologics. However, over the past decade, LC–MS/MS has been gaining in popularity and acceptance as a viable alternative technique for the quantitation of protein therapeutics.
An important facet of developing a robust chromatographic bioanalytical method that is capable of producing accurate and precise data is identification and selection of an appropriate internal standard. The ideal internal standard should have chemical and physical properties that match or are nearly identical to those of the target analyte. MS-based detection allows the use of stable isotope-labeled analogs as internal standards, which ideally co-chromatograph with the unlabeled analyte and are simultaneously measurable based on mass (mass/charge) selectivity. For protein-based methods there are several types of internal standards that are most commonly utilized: stable isotope-labeled intact protein (SIL-protein), stable isotope-labeled peptide (SIL-peptide), and stable isotope-labeled extended peptides (extended SIL-peptide). The use of each type of internal standard will be discussed with the main focus on protein therapeutics that are too large for practical direct analysis using LC-MS/MS technology. These molecules typically require digestion to yield an appropriate signature peptide that is measurable as a surrogate for the intact protein.
SIL-protein internal standards
The ideal internal standard would be a SIL-version (or isotopologue) of the protein therapeutic itself, as it would have virtually identical properties to the therapeutic being measured, and would compensate for enrichment and digestion variations [2]. To illustrate, our laboratory was developing an assay to measure total antibody concentrations and antibody–conjugated drug concentrations from a proprietary antibody–drug conjugate (ADC). The ADC was enriched using immunocapture and subsequently digested with trypsin, producing surrogate peptides for quantitating the total antibody concentration and the (deconjugated) drug for quantitating the antibody–conjugated drug concentration. A SIL-mAb was used as an internal standard for measuring total antibody concentrations, and stable isotope-labeled drug, analogous to using a SIL-peptide, was used as the internal standard for measuring the antibody-conjugated drug concentrations. The SIL-mAb was added prior to the immunocapture step while the SIL-drug was added after the digestion. During development, the precision and accuracy for the total antibody concentrations were typically ≤10%, however the antibody–conjugated drug concentrations had much higher variability. The SIL-mAb compensated for variations in the antibody capture and digestion steps as quantitation based on surrogate tryptic peptides was not impacted for total antibody; however, the SIL-drug did not participate in the capture and digestion steps, thus leading to uncompensated variability for the antibody–conjugated drug. The observed issue was later found to be related to variation in the deconjugation process occurring during the trypsin digestion, which was subsequently corrected.
Solution-related problems are often encountered with proteins and peptides, which have a propensity towards non-specific binding (NSB) to container surfaces when prepared in neat aqueous-based solutions devoid of protein and a tendency to denature or precipitate when exposed to organic solvents. An effective way to minimize NSB is to add a protein such as bovine serum albumin (BSA). Therefore, SIL-protein internal standard solutions are often prepared in phosphate buffered saline (PBS) with 0.5 to 5% BSA or directly in biological matrix. Since SIL-protein internal standards are added prior to sample preparation, the additional BSA is typically removed during the enrichment procedure and has little to no impact on the assay.
Although SIL-proteins are the ideal internal standard, the complexity, time and cost to produce them using recombinant synthesis often limit their availability. As such, suitable alternative internal standards are often required, which typically involve the use of SIL-peptides.
SIL-peptide internal standards
From a time and cost perspective, SIL-peptide internal standards (ISs) can be chemically synthesized relatively quickly (~3 weeks) and cost effectively by many commercial vendors. Using a SIL-peptide internal standard requires it to be added after the enrichment step, for example, after immunocapture, protein precipitation and albumin depletion because it would otherwise be discarded via washing or during aspiration of the supernatant. As described above, proteins and peptides are susceptible to NSB when prepared as neat solutions. Since the SIL-peptides are often added after the enrichment step (i.e., following removal of endogenous proteins), preparing SIL-peptides in a modified protein solution could have a significant impact on the assay performance as unwanted proteins would be added back into the sample. The SIL-peptide diluent is often a weak organic water mixture, for example 80:20 water/acetonitrile, and the diluent’s impact on NSB or SIL-peptide solubility, as well as the digestion and chromatographic performance of the assay, should be carefully evaluated. More importantly, it should be kept in mind that SIL-peptide ISs are unable to compensate for variability associated with most of the sample preparartion steps, and thorough optimization of the enrichment and digestion conditions is needed. In our laboratory, even after careful optimization, we have observed an increase in variability when performing a large batch that spans two 96-well plates. Typically, a batch contains, at a minimum, duplicate calibration curves and duplicate run acceptance QCs. When analyzing a two-plate batch the calibration curves and QCs are split across the two plates, with one complete curve on each plate. The within plate variability is often acceptable; however, the variability between plates results in divergent calibration curves (i.e., with different slopes) leading to a potential batch failure. Although the enrichment and digestion efficiencies are typically very reproducible within a single 96-well plate, slight differences in efficiencies between plates can result in divergent curves. Therefore, we typically avoid performing single-batch runs that span two 96-well plates when using SIL-peptide internal standards. Instead, two one-plate self-contained batches would be performed to avoid this situation with minimal impact on daily throughput.
Additionally, an evaluation should be performed to determine the most appropriate post-enrichment step to add the SIL-peptide. The typical post-enrichment steps involve denaturing, reducing, alkylating and digesting with an appropriate enzyme, which can have different stability characteristics for a naked peptide versus intact protein. To illustrate, our laboratory was developing a proprietary method for a fusion protein, wherein a SIL-peptide IS was added after the immunocapture step but prior to digestion. The signature peptide from digestion of the protein had good recovery but the SIL-peptide internal standard had extremely low recovery. After careful evaluation, the stability of the SIL-peptide was found to be negatively impacted by the buffer pH, time, and temperature used for the digestion, whereas the signature peptide from the protein was nearly unaffected. Therefore, to overcome the observed stability differences between the signature peptide from the protein therapeutic and the naked SIL-peptide IS, the SIL-peptide IS was added after the enzyme digestion was complete. While SIL-peptides may not be the ideal internal standard due to lack of tracking enrichment and digestion, they have been successfully used within our laboratory and by many others in the scientific community [3–5] and have the additional advantage of being easy to obtain and relatively inexpensive.
Extended SIL-Peptide Internal Standards
Extended SIL-peptides, typically with at least four amino acids added to both the N- and C-terminus, offer similar time and cost advantages as SIL-peptides, with the additional advantage of being able to potentially compensate for digestion variability. However, extended SIL-peptides have the same disadvantage as SIL-peptides in that they cannot compensate for enrichment variations. An example of the added benefits of using extended SIL-peptides was reported by Faria et al.[6]. In this work it was shown that the signature peptide was susceptible to degradation attributed to chymotrypsin-like activity assumed to arise from trypsin autolysis. Since the protein was being digested to the signature peptide, there was a time delay related to its formation (formation phase) followed by degradation (degradation phase), which were occurring at the same time. This presented a potential problem for using a SIL-peptide as it did not require a formation phase. As such the SIL-peptide and signature peptide degradation rates did not match leading to variability. The extended SIL-peptide had a formation phase and degradation phase that closely matched that of the intact protein, thus minimizing the apparent digestion variability.
The appropriate number of flanking N- and C-terminal amino acids that are needed to track the digestion of the whole protein is not easy to predict. Digestion of the extended SIL-peptide may be either too quick and easy or more variable and even incomplete. In our laboratory we had an extended SIL-peptide synthesized with four amino acids added to both the N- and C-terminus. The trypsin digestion recovery of the protein and extended SIL-peptide was evaluated by comparing to an unlabeled synthetic version of the surrogate peptide. The digestion recovery associated with the protein was estimated to be approximately 75%; however, the recovery for the extended SIL-peptide was only approximately 15%. This vast difference was attributed to a significant reduction in enzyme activity towards the extended SIL-peptide due to the limited number of amino acids flanking the digestion sites.
Analog SIL-protein for use as a surrogate for monitoring enrichment and digestion
As discussed above, SIL-protein internal standard availability may be limited and the use of SIL-peptide or extended SIL-peptide internal standards do not compensate for enrichment and/or digestion. Our laboratory is evaluating a combination approach using a SIL-peptide internal standard and an analog SIL-protein for extra monitoring purposes. To illustrate, we are developing an immunocapture LC-MS/MS assay for a monoclonal antibody therapeutic, which measures a signature peptide from the CDR. Since an ideal SIL-protein therapeutic internal standard is not available, we are using SIL-peptide internal standards added after the capture and digestion steps to perform quantitation. We are also adding a commercial SIL-mAb as a surrogate intact antibody protein prior to enrichment to monitor general capture and digestion efficiency. A SIL-peptide from the SIL-mAb framework will be measured and its response across the run evaluated for sample-to-sample consistency. It’s important to note that during method validation, all samples are known samples and if a problem arises during the capture and or digestion steps, it is relatively easy to detect. However, for unknown samples, problems with the capture or digestion are not necessarily easy to identify as the SIL-peptide IS responses would appear normal. Adding the SIL-mAb provides additional information pertaining to both the capture and digestion and can be used to help identify or troubleshoot potential aberrant unknown sample concentrations. While the SIL-mAb does not produce appropriate peptides for use as an internal standard for quantification, it does provide a means for monitoring the overall capture and digestion consistency.
In closing, the choice of internal standard is often dictated based upon availability, time and cost. While SIL-proteins are considered the ideal internal standard, their availability often limits their use. However, SIL-peptides and extended SIL-peptides are readily available, and serve as good alternatives to SIL-proteins, provided that solution preparation/handling, and sample preparation enrichment and digestion steps are characterized and thoroughly evaluated. The use of analog SIL-proteins, in combination with SIL peptide internal standards, particularly for mAb-based therapeutics, has the promise of adding an additional level of confidence to the analysis of unknown samples as the enrichment and digestion consistency could be evaluated on a sample-by-sample basis.
References
- Biologic Therapeutic Drugs: Technologies and Global Markets. BCC Research (2015).
- Furlong M, Zhao S, Mylott W et al. Dual universal peptide approach to bioanalysis of human monoclonal antibody protein drug candidates in animal studies. Bioanalysis 5(11), 1363 – 1376 (2013).
- An B, Zhang M, Johnson R, Qu J. Surfactant-aided precipitation/on-pellet-digestion (SOD) procedure provides robust and rapid sample preparation for reproducible, accurate and sensitive LC/MS quantification of therapeutic protein in plasma and tissue. Chem. 87, 4023 – 4029 (2015).
- Zhao Y, Guowen L, Angeles A et al. Development and validation of a liquid chomatogrpahy tandem mass spectrometry assay for the quantitation of a protein therapeutic in cynomolgus monkey serum. Chromatogr. B 988, 81-87 (2015).
- Tang W, Kernstock R, Simmons N, Alak A. Elisa microplate: a viable immunocapture platform over magnetic beads for immunoaffinity-LC-MS/MS quantitation of protein therapeutics?. Bioanalysis 7(3), 307 – 318 (2015).
- Faria M, Halquist M, Yuan M, Mylott W, Jenkins R, Karnes H. Comparison of a stable isotope labeled (SIL peptide and an extended SIL peptide as internal standards to track digestion variability of an unstable signature peptide during quantification of a cancer biomarker, human osteopontin, from plasma using capillary microflow LC-MS/MS. Chromatogr. B 1001, 156 – 68 (2015).