Recent advances in bioanalysis and metabolite profiling of oligonucleotide therapeutics
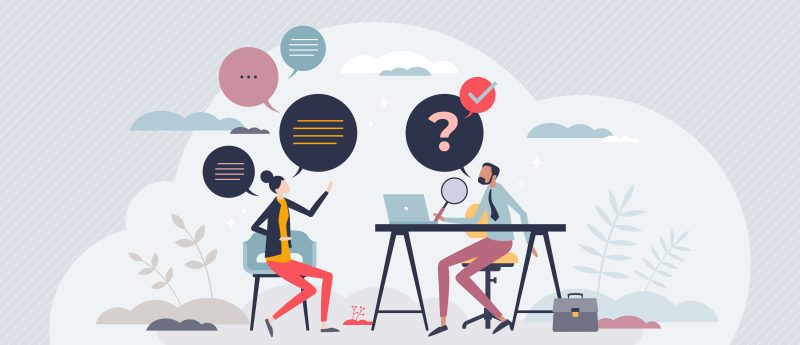
Laixin Wang, Co-founder and Senior Vice President of Chongqing Denali Medpharma Co., Ltd. (China) obtained his PhD in Medicinal Chemistry from Beijing Medical University (now Peking University, China). He subsequently conducted post-doctoral training in Pharmaceutical Chemistry and Combinatory Chemistry in University Utah (UT, USA) and Duke University (NC, USA). Dr Wang started his industry career as a senior research scientist working on formulation and delivery of oligonucleotide therapeutics and hydrophobic drugs in a startup biotech company. After the company was acquired, Dr Wang joined Tandem Labs (now Covance; VA, USA) supporting GLP bioanalysis and dose formulation analysis. He assumed increasing leadership roles for managing scientists and projects in the quantitation of both small and large molecular therapeutics/biomarkers using LC–MS/MS, LC–HRAM, UPLC-UV and hybridization-based LC-fluorescence assays. He was an associate laboratory director at Tandem Labs (UT, USA) prior to joining NovaBioAssay (MA, USA) as the Vice President of bioanalytical in 2015. Dr Wang returned to China to co-start the Chongqing Denali Medpharma Co. and Chongqing Helens Clinical Research Center Co. in 2017.
The development of oligonucleotide-based therapeutics has gone through development cycles, ranging from intense excitement to abandonment and then rediscovered enthusiasm as the technology evolved and the previous challenges and resulting failures were overcome. Throughout these cycles, there has been steady progress in advancing the technologies. The recent approvals of several antisense and siRNA provide optimism that oligonucleotide-based therapeutics will continue to emerge as an important class of therapeutics [1,2].
Oligonucleotides can interfere with biomolecules representing the entire extended central dogma. Up to now, seven oligonucleotide-based drugs have received market authorization by regulatory authorities. All of them are chemically modified oligonucleotides containing 18–30 nucleoside units. The chemical modification can affect pairing, stability and conformation of the oligonucleotides, and therefore affect their interactions with proteins and either RNA or DNA. Improved knowledge on nucleic acid chemistry and RNA/protein regulatory mechanisms are leading to the design and development of more potent and convenient oligonucleotide therapeutics. The evolving applications and reduced dosages to be effective inevitably generate an increased demand for sensitive, accurate and reliable methods for qualitative and quantitative analysis of oligonucleotides in a variety of biological matrices.
This editorial will briefly discuss the recent advances in the techniques for bioanalysis of oligonucleotide therapeutics with the focus on novel hybridization-ELISA and chromatographic-based assays that are simple and suitable for all chemically modified oligonucleotide therapeutics.
Meso Scale Discovery electrochemiluminescence (MSD-ECL) ELISA
MSD-ECL ELISA is a method similar to conventional ELISA except that MSD uses electrochemiluminescence (ECL) as a detection technique as opposed to a colorimetric reaction in plates employed by conventional ELISA. An ECL system such as the MSD platform has many advantages over a traditional ELISA system such as: higher sensitivity, increased dynamic range (up to 5 orders of magnitude), less matrix effects, ability to multiplex and less sample volume required [3, 4]. The only drawback is that the assays are still not able to distinguish the parent drugs from their critical metabolites. Therefore, the MSD-ECL ELISA is gaining popularity for quantitative analysis of oligonucleotide therapeutics to support the studies that require very sensitive assays. The use of locked nucleic acid (LNA) modified DNA probes can further improve the assay sensitivity. Combining the LNA probe design and MSD ECL detection, Thayer et al. developed an assay with a dynamic range of 0.1–10,000 pM in serum, liver and kidney homogenate for the detection of a 22-mer modified siRNA [4].
Enzyme-linked oligonucleotide hybridization assay
Enzyme-linked oligonucleotide hybridization assays (ELOHA) are based on the competition of analytes with a detection oligo for hybridization to a capture oligo covalently linked to a solid substrate. The versatility of ELOHA was recently demonstrated by Lorenson et al. using the measurement of three oligos, including two 25-mer morpholino-oligomers and an unmodified 23-mer DNA oligonucleotide [5]. The assays have sensitivity as low as 0.28 pmol/sample reaction at 50% hybridization. Unlike the MSD-ECL-ELISA which requires a proprietary instrument, these assays only require a basic 96-well plate colorimetric reader which is standard in clinical and research laboratories.
Triplex-forming oligonucleotide (TFO) ELISA for quantitation of siRNA-protein conjugates
Triplex-forming oligonucleotide (TFO) ELISA was developed to quantify intact double-stranded siRNA complexes. It is well known that appropriately designed LNA or peptide nucleic acid (PNA) containing probes can form stable triplexes with their target DNA or RNA duplexes for a variety of biomedical applications [6]. Based on this mechanism, Humphreys et al. developed a TFO-ELISA to quantify intact siRNA-mAb conjugates in biological matrices [7]. In their preliminary studies, a lower LOD of 120 pg/mL was achieved to detect intact siRNA-protein conjugates in serum or plasma. With further characterization and optimization of the LNA modification pattern in the TFO, it is believed that the assay sensitivity could be further improved. Although this is the first study providing a proof of concept for the application of TFOs to detect intact siRNA-mAb conjugates, this technology can be readily applied to other modalities, including siRNA conjugated to peptides or other non-mAb proteins, unconjugated siRNA, or polynucleotides in bioanalytical samples.
Liquid chromatography coupled high-resolution accuracy mass spectrometry (LC-HRAM) for sensitive and accurate quantitation and metabolite profiling
Liquid chromatography coupled with mass spectrometry technologies (LC–MS) have become important analytical tools for the analysis of oligonucleotides because of their capabilities for both accurate identification and quantification. Many attempts have been devoted to improve the assay sensitivity, specificity and throughput by improving sample preparation, LC separation and MS technologies.
1. Sample extraction
Sample cleanup is a critical step to removing most of the lipids and proteins in biological matrices for LC–MS analysis of oligonucleotides. While liquid–liquid extraction (LLE) is still proven to be the most effective extraction method for plasma and urine assays, several solid phase extraction (SPE) methods or the combination of LLE and SPE methods have been developed for various structures of oligonucleotides in a variety of biological matrices [8–13]. Unlike LLE, which is simple and works for almost all classes (except for the short hydrophobic oligomers) of oligonucleotides, SPE is very sensitive to the sequences and chemical modifications of the target oligonucleotides. Therefore, the SPE sorbents and/or extraction conditions need to be carefully selected and optimized for each individual class of oligonucleotide therapeutics. We found it is particularly challenging to extract hydrophobic group modified oligonucleotides from biological matrices. Therefore, the probe hybridization extraction method is probably the preferred option for LC–MS analysis [9].
2. Liquid chromatographic separation
Many chromatographic separation methods have been used for the analysis of oligonucleotides including anion-exchange chromatography, hydrophilic interaction chromatography (HILIC) and ion-pair reversed-phase liquid chromatography (IP-LC). However, IP-LC is still the major technique used for LC–MS analysis of oligonucleotide therapeutics [14]. The ion pairing reagents and the mobile phase compositions for the analysis of a variety of oligonucleotides were extensively studied by different research groups over the last few years [15–17]. The 1,1,1,3,3,3- hexafluoro-2-propanol (HFIP) and trimethylamine (TEA) or N,N-Diisopropylethylamine (DIPEA) together with methanol remain the most effective mobile phases for a majority of the therapeutic oligonucleotides. However, in some cases, other fluoroalcohol and/or alkylamine combinations may provide better sensitivity and/or separation depending on the length, sequence and chemical modifications of the oligonucleotides. The advances in column chemistry and packing techniques are also providing more durable and higher resolution LC columns for IP separation of oligonucleotides. One example is the new wide-pore hydrophobic polymer based DNAPac RP column [18]. These columns can tolerate high pH and/or high temperature conditions, and provide excellent separation for a variety of single- and double-stranded oligonucleotides under MS compatible conditions. More importantly it has an increased life time and lower carry-over which are critical for bioanalysis of oligonucleotide therapeutics.
In addition to IP-LC, HILIC is advancing to a promising alternative for the analysis of oligonucleotides [19]. Instead of the hydrophobic stationary phase employed in IP-LC, HILIC stationary phases consist of polar (hydrophilic) stationary phases where compounds are eluted in order of increasing polarity. Like RP-LC columns, most of the stationary phases in HILIC columns are silica based. These include those as simple as bare silica or bonded phases that contain neutral or ionizable functional groups. But unlike most RP separations, there is no single HILIC stationary phase that can be universally applied to the separation of polar compounds. Without the use of ion-pairing reagents, MS ionization of oligonucleotides in HILIC conditions is proven to be more efficient compared to IP-RPLC conditions. However, early studies were not able to achieve satisfactory LC separations for complex mixtures of oligonucleotides [17]. More recently, a new polymer-based diol HILIC column (Shodex VN-520) was reported to produce good separation and sensitivity for LC–MS/MS analysis of a variety of oligonucleotides in biological matrices under carefully optimized mobile phase compositions and gradient conditions [19]. Additionally, a direct comparison experiment demonstrates that the HILIC-based LC–MS methods yielded comparable sensitivities and separations to existing IP-RP-LC methods for various classes of oligonucleotide samples, providing the basis for alternative options for sensitive, reproducible and quantitative analysis of oligonucleotides without the need for ion-pair reagents
3. Mass spectrometry detection
The development of sensitive LC–MS/MS assays remains challenging for oligonucleotides despite the substantial improvements recently identified in sample extraction, liquid chromatographic separations and mass spectrometry detection methodologies. Assays with sub-ng/mL LLOQ were only reported for measuring some short oligonucleotides in plasma with well-designed sample extraction and LC–MS analysis conditions [13, 20]. Notably, high-resolution accurate-mass (HRAM) mass spectrometry appears to achieve comparable or better sensitivity for most of the evaluated therapeutic oligonucleotides and therefore are gaining increasing applications in their bioanalysis for the non-phosphorothioate containing oligonucleotides [12]. For the quantitation of phosphorothioate modified oligonucleotides, LC–MS/MS methods are sometimes advantageous on a triple quadrupole MS as the major and unique fragmentation PO2S– (m/z= 94.9) ion provides sensitive and adequately specific quantitation [17, 21]. However, to enable the distinction between PO2S– (m/z= 94.9362) and PO4– (m/z=94.9540) ions, a minimum resolving power of 5500 would be required. This resolution is not feasible for current triple quadrupole MS systems but can be easily achieved using either Orbitrap or TOF mass analyzers. Actually, by monitoring the PO2S– product ion of a 22-mer phosphorothioate oligonucleotide using high-resolution multiple reaction monitoring (MRMHR) on a TripleTOF® 6600 System, Tozaki et al. were able to develop an assay to successfully quantify a 22-mer phosphorothioate oligonucleotide in plasma with a dynamic range of 0.1–200 ng/mL in horse plasma [20]. Another big advantage for LC-HRAM-MS analysis is that typical m/z peaks or MS/MS transitions for particular analytes are not required to be selected prior to the data acquisition making it possible for simultaneously quantitative and qualitative analysis of oligonucleotide therapeutics and their metabolites in biological matrices [22]. Additionally, the LC-HRAM-MS utility for the rapid determination of the relative amounts of coeluting impurities in oligonucleotide product samples has been demonstrated [23].
Hybridization LC–fluorescence assay for sensitive and specific quantitation of modified oligonucleotides
Hybridization LC–fluorescence assays are the combination of probe hybridization and LC–fluorescence analysis. These hybrid assays maintain the advantages of both types of assays. Similar to hybridization ELISA assays, hybridization LC–fluorescence assays can easily achieve a 1.0 ng/mL or lower LLOQ to quantify oligonucleotides in plasma or tissue homogenate without the use of special sample extractions [24,25]. Parent drugs can be separated adequately via liquid chromatography to distinguish them from their critical metabolites, including the separation between 5΄-phosphorylated and non-phosphorylated guide strands of the siRNA [26]. Additionally, since no enzyme is involved in the entire process, the assays are not affected by oligonucleotide structural modifications like lipid group conjugations [25]. The only challenge is the requirement of an appropriate probe design which is critical for assay sensitivity, specificity and dynamic range. This leads to longer method development times in addition to increased cost [27]. Therefore, these assays are usually used to support clinical or late stage preclinical studies, while the LC-HRAM-MS assays are more commonly used in drug discovery or early development stages.
Discussion and future perspectives
As non-modified DNA or RNA oligonucleotides are not stable in biological matrices and cannot adequately penetrate into living cells, the therapeutic oligonucleotides are usually chemically modified to reach their target and produce the desired pharmacological effects. Although qPCR is a golden standard for sensitive quantitation of DNA and mRNA molecules, the assays usually have insufficient sensitivity for short or heavily modified oligonucleotide therapeutics because qPCR bioanalysis of therapeutic oligonucleotides involves sample extraction and PCR amplification. Additionally, they require expensive reagents, complex instrumentation and careful assay optimization [5, 28]. MSD-ECL-ELISA and ELOHAs do not require extensive plasma/tissue extractions, they have better sensitivity compared to conventional hybridization ELISA and their assay dynamic ranges are significantly improved. The only drawback is that these assays are being unable to distinguish the full length analytes from their close metabolites. As a complementary technique, the LC–MS assay can clearly distinguish the analyte oligonucleotides from their metabolites. However, the development of sensitive LC–MS/MS assays remains challenging. Lowering the limit of quantification to 1 ng/mL or below still appears difficult with the exception of some short oligonucleotides. Given they are easy to use and usually more sensitive compare to conventional LC–MS/MS assays, LC-HRAM-MS assays have shown many advantages in supporting research and development of oligonucleotide therapeutics. More importantly, they can be used to conduct quantitative analysis and metabolite profiling simultaneously. Hybridization LC–fluorescence assays are good compromises between the ECL-ELISA and LC–MS methods. They are adequately sensitive and specific for most of the oligonucleotide therapeutics. As the fluorescent probes need to be carefully designed and optimized for each specific oligonucleotide analyte, they are often used to support late stage preclinical research of clinical studies.
[1] Smith CIE & Zain R. Therapeutic oligonucleotides: state of the art. Rev. Pharmacol. Toxicol. 59, 25.1–25.26 (2019).
[2] Huang Y. Preclinicaland clinical advances of GalNAc decorated nucleic acid therapeutics. Mol. Ther. Nucleic Acids. 17(6), 116–132 (2017).
[3] Shemesh CS, Yu RZ, Gaus HJ et al. Pharmacokinetic and pharmacodynamic investigations of ION-353382, a model antisense oligonucleotide: using alpha-2-macroglobulin and murinoglobulin double-knockout mice. Nucleic Acid Ther. 26(4), 223–235 (2016).
[4] Thayer MB, Lade JM, Doherty D et al. Application of locked nucleic acid oligonucleotides for siRNA preclinical bioanalytics. Report, 9(1), 3566 (2019).
[5] Lorenson MY, Chen KHE & Walker. Enzyme-linked oligonucleotide hybridization assay for direct oligo measurement in blood. Biology Methods and Protocols. 4(1), 1–8 (2019).
[6] Gupta A, Mishra A & Puri N. Peptide nucleic acids: advanced tools for biomedical applications. Biotechnol. 259, 148–159 (2017).
[7] Humphreys SC, Thayer MB, Campuzano IDG, Netirojjanakul C & Rock BM. Quantification of siRNA-antibody conjugates in biological matrices by triplex-forming oligonucleotide ELISA. Nucleic Acid Ther. doi: 10.1089/nat.2018.0770 (2019) (Epub ahead of print).
[8] Franzoni S, Vezzelli A, Turtoro A et al. Development and validation of a bioanalytical method for quantification of LNA-i-miR-u221, a 13-mer oligonucleotide, in rat plasma using LC–MS/MS. Pharm. Biomed. Anal. 150, 300–307 (2018).
[9] Dillen L, Sips L, Greway T & Verhaeghe T. Quantitative analysis of imetelstat in plasma with LC–MS/MS using solid-phase or hybridization extraction. Bioanalysis. 9(23), 1859–1872 (2017).
[10] Meng M, Zhang J, Liu A, Reuschel S, Sazani P & Wong M. Quantitative determination of AVI-7100 (Radavirsen), a phosphorodiamidate morpholino oligomer (PMOplus®), in human plasma using LC–MS/MS. Bioanalysis. 9(10), 827–839 (2017).
[11] Ewles M, Goodwin L, Schneider A & Rothhammer-Hampl T. Quantification of oligonucleotides by LC–MS/MS: the challenges of quantifying a phosphorothioate oligonucleotide and multiple metabolites. Bioanalysis. 6(4), 447–64 (2014).
[12] Wheller R, Summerfield S & Barfield M. Comparison of accurate mass LC–MS and MRM LC–MS/MS for the quantification of a therapeutic small interfering RNA. Int. J. Mass Spectrom. 345–347, 45–53 (2013).
[13] Hemsley M, Ewles M & Goodwin L. Development of a bioanalytical method for quantification of a 15-mer oligonucleotide at sub-ng/ml concentrations using LC–MS/MS. Bioanalysis. 4(12), 1457–69 (2012).
[14] Kaczmarkiewicz A, Nuckowski Ł, Studzińska S & Buszewski B. Analysis of antisense oligonucleotides and their metabolites with the use of ion pair reversed-phase liquid chromatography coupled with mass spectrometry. Rev. Anal. Chem. doi: 10.1080/10408347.2018.1517034 (2019) (Epub ahead of print).
[15] Liu R, Ruan Y, Liu Z & Gong L. The role of fluoroalcohols as counter anions for ion-pairing reversed-phase liquid chromatography/high-resolution electrospray ionization mass spectrometry analysis of oligonucleotides. Rapid Commun. Mass Spectrom. 33(7), 697–709 (2019).
[16] Li N, El Zahar NM, Saad JG, van der Hage ERE & Bartlett MG. Alkylamine ion-pairing reagents and the chromatographic separation of oligonucleotides. Chromatogr. A. 1580, 110–119 (2018).
[17] Studzińska S, Rola R & Buszewski B. The impact of ion-pairing reagents on the selectivity and sensitivity in the analysis of modified oligonucleotides in serum samples by liquid chromatography coupled with tandem mass spectrometry. Pharm. Biomed. Anal. 138, 146–152 (2017).
[18] Baek J, Thayer J, Lin SH, Birznieks I, D’Silva K & Cubbon S. Chromatography for the analysis of oligonucleotides. Chromatography Today, 10(2), 26–29 (2017).
[19] Lobue PA, Jora M, Addepalli B & Limbach PA. Oligonucleotide analysis by hydrophilic interaction liquid chromatography-mass spectrometry in the absence of ion-pair reagents. Chromatogr. A. pii: S0021-9673(19)30146-3 (2019) (Epub ahead of print).
[20] Tozaki T, Karasawa K, Minamijima Y et al. Detection of phosphorothioated (PS) oligonucleotides in horse plasma using a product ion (m/z 94.9362) derived from the PS moiety for doping control. BMC Res. Notes. 11(1), 770 (2018).
[21] Husser C, Brink A, Zell M, Müller MB, Koller E & Schadt S. Identification of GalNAc-conjugated antisense oligonucleotide metabolites using an untargeted and generic approach based on high resolution mass spectrometry. Chem. 89(12), 6821–6826 (2017).
[22] Friese OV, Sperry JB, He Y, Joseph L, Carroll JA & Rouse JC. Characterization of apolipoprotein C3 (Apo C3) LNA/DNA impurities and degradation products by LC–MS/MS. AAPS J. 19(6):1735–1744 (2017).
[23] Roussis SG, Cedillo I & Rentel C. Semi-quantitative determination of co-eluting impurities in oligonucleotide drugs using ion-pair reversed-phase liquid chromatography mass spectrometry. Chromatogr. A. 1584, 106–114 (2019).
[24] Tian Q, Rogness J, Meng M & Li Z. Quantitative determination of a siRNA (AD00370) in rat plasma using peptide nucleic acid probe and HPLC with fluorescence detection. Bioanalysis. 9(11), 861–872 (2017).
[25] Godinho BMDC, Gilbert JW, Haraszti RA et al. Pharmacokinetic profiling of conjugated therapeutic oligonucleotides: a high-throughput method based upon serial blood microsampling coupled to peptide nucleic acid hybridization assay. Nucleic Acid Ther. 27(6), 323–334 (2017).
[26] Trubetskoy VS, Griffin JB, Nicholas AL et al. Phosphorylation-specific status of RNAi triggers in pharmacokinetic and biodistribution analyses. Nucleic Acids Res. 17, 45(3), 1469–1478 (2017).
[27] Ji YH, Liu YJ, Xia WH, Behling A. Bennett P & Wang L. Probe design is critical for the sensitivity and specificity of the bioanalysis of oligonucleotide using hybridization based LC–fluorescence assays. Bioanalysis, 2019, to be published.
[28] Wang L & Ji C. Advances in quantitative bioanalysis of oligonucleotide biomarkers and therapeutics. Bioanalysis. 8(2), 143–55 (2016).
Our expert opinion collection provides you with in-depth articles written by authors from across the field of bioanalysis. Our expert opinions are perfect for those wanting a comprehensive, written review of a topic or looking for perspective pieces from our regular contributors.
See an article that catches your eye? Read any of our articles below for free.