Quantitative High Resolution Mass Spectrometry Coming of Age
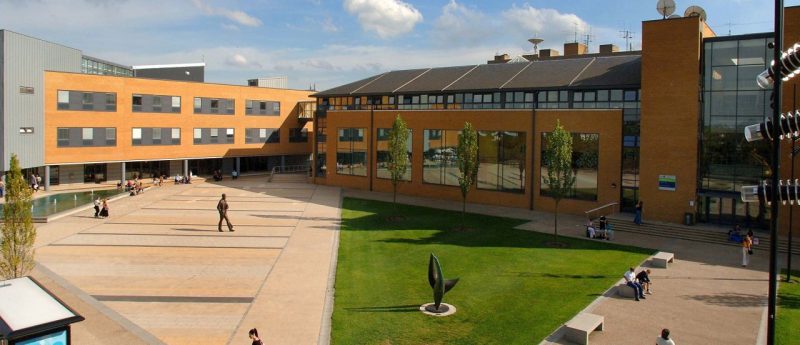
Introduction
High Resolution Mass Spectrometry (HRMS) is a powerful technique that has been primarily applied to qualitative discovery analysis such as structural characterization of biopharmaceuticals, identification of metabolites as well as proteomics and metabolomics.
In addition to its powerful applications in qualitative analysis, recent advances in instrumentation have enabled application of HRMS to quantitative bioanalytical workflows in both high resolution tandem mass spectrometry (MS/MSHR) or full scan modes which open new horizons in quantitative bioanalysis. Herein we will cover the relevant HRMS instrumentation, discuss the advantages and disadvantages of HRMS as applied to quantitative workflows and provide examples of its applications. We will also examine HRMS role in regulated bioanalysis.
Instrumentation
There are several types of mass analyzers that have the ability to generate high resolution data.
Fourier transform ion cyclotron resonance (FT-ICR) instruments are state-of-art and offer extraordinary ability to generate spectra with resolution up to 1,000,000 and thus unambiguously determine chemical formulae of compounds of interest from isotopic fine structure. However, FT-ICR is still usually used for discovery or characterization research [1]. The low scanning speed makes FT-ICR incompatible with most chromatographic separations. In addition, the high cost, bulky size and difficult maintenance limit the utility of FT-ICR to specialized applications.
Orbitrap is a subtype of FT-ICR instrument class. Unlike with traditional FT-ICR, orbitrap’s ability to generate high resolution data (up to 200,000 resolution) with minimal maintenance, less cost and the smaller size has greatly facilitated its broader application. Additionally, orbitraps have improved scan rates that can reach 60,000-100,000 resolution at 1Hz or 10,000 at 10Hz. For a UPLC peak width of 5-20 seconds, this scan speed is on the edge of generating sufficient number of points to enable quantitative assessment. Several different types of orbitrap instruments offered by Thermo Fischer Scientific have been applied to quantitative analysis (Velos-Orbitrap, Q-Exactive and Orbitrap Fusion) [2-4].
Time of flight (TOF) instruments offer moderate mass resolution (up to 50,000) and flexible scan rate on the time scale appropriate for quantification with liquid chromatography (LC) separation. Instruments such as Q-TOF and TripleTOF from Waters, Agilent, Sciex and Bruker [5, 6] have been reported to be used for quantitative assays.
Recent advances in HRMS instrumentation such as improved sensitivity and dynamic range have expanded its application to quantitative workflows. However, as high resolution is not the only consideration for mass spectrometry assay development it should be considered to suit the objectives of each project. Additional considerations include: scan rate, up front separation, sample complexity and the analytical question at hand.
Advantages
HRMS instruments have the ability to determine accurate mass and isotopic distribution compared to triple quadrupole (QQQ) instruments. When applied to quantification, significant and sometimes critical advantages exist for HRMS.
High resolution and mass accuracy data enable high selectivity and specificity. Compounds with close mass that may overlap in a QQQ instrument will show a clear difference in the HRMS [7]. The rich information obtained utilizing HRMS provide more freedom to analyze for metabolites/biotransformations with small mass change, or to simply eliminate the back ground noise and increase the sensitivity. This becomes even more important when analyzing multiply charged ions, where the m/z difference between compounds is smaller.
High resolution data in full scan mode can provide sufficient specificity and selectivity, so that it can be used for quantification without product ion confirmation. This allows faster method development time with minimal need for compound optimization and is especially helpful for analyses involving multiple targets. Obtaining full scan data also allows for revisiting the data for additional analytes which is particularly useful for biomarker discovery and metabolite identification (met ID) workflows and enables assay multiplexing [7].
When used in product ion mode, HRMS has the possibility to record the full tandem mass spectrum in high resolution and sum multiple ions for obtaining extracted ion chromatograms (XIC). This increases the sensitivity of the HRMS and can be beneficial for compounds with complex fragmentation patterns.
HRMS instruments are often used as the discovery instrument from the beginning of a project; therefore, method development for a quantification assay with HRMS can be faster.
Disadvantages
However, some challenges still exist that limit broader utilization of HRMS instruments in quantitative bioanalysis:
- Cost is still a significant factor in obtaining HRMS instruments, although it has been significantly reduced, the price of a HRMS instrument is often twice or more compared to QQQ or single quadrupole instruments.
- Instrument maintenance for HRMS is more complex compared to a standard QQQ. While front end cleaning is required for all instruments, HRMS need to be regularly calibrated to maintain the high mass accuracy and resolution. TOF instruments in particular need to have frequent calibration during the run sequence for good mass accuracy. Therefore more effort is needed to ensure method robustness. Automatic calibrant delivery systems significantly help facilitate this process.
- One common consideration for bioanalytical methods is the simplicity of method transfer. While the instrument companies are making effort to use similar ionization sources within their product lines and similar acquisition software, the difference in dynamic range and detectors might generate a method transfer gap that needs to be addressed with further effort. Additionally, lack of familiarity with instrumentation/method development of quantitative assays using HRMS for many users still requires significant training.
- Data file storage can also become a serious concern when using HRMS in full scan mode for large numbers of samples. Thus, acquisition of many samples that may never be mined in the future could be a significant obstacle for implementing HRMS for large studies [8]. Data compression can address some of these concerns.
- Additionally, in case of regulated bioanalysis, it is important to that the study protocol (and in case of clinical studies also the informed consent form) enable such data exploration.
Applications
One application for quantitative HRMS (qHRMS) is the ability to provide more quantitative information for various “omics” workflows such as proteomics or metabolomics [9-12]. HRMS significantly reduces the ambiguity in the assignment of the detected analytes due to isobaric interferences present in complex samples. Additionally, improved sensitivity and dynamic range of modern HRMS instruments enable extraction of quantitative data from runs acquired in full scan mode. This application is of particular advantage for clinical studies where due to limited availability it might not be possible to re-interrogate samples for a targeted analysis on a QQQ instrument following a discovery run on an HRMS one. Thus, ability to extract quantitative information from full scan HRMS analysis is critical for assessment of rare/low abundance samples. Additionally MS/MSHR also offers the ability to quantify sets pre-defined analytes for both relative and absolute quantification in combination with appropriate internal standards [13, 14].
As mentioned above, qHRMS can be advantageous for metID studies of both small molecules and biopharmaceuticals where one can extract quantitative information for many metabolites from a single full scan run while still obtaining quantitative information enabling pharmacokinetic analyses [15]. This would be particularly important for situations where novel metabolic pathways may play a role and lead to discovery of unexpected metabolites. Alternatively one could consider employing MS/MSHR for rapid quantification of a number of metabolites of interest without investing significant amounts of time in method optimization. Additionally, recent developments in software facilitate high throughput metID workflows using HRMS [16].
One example where qHRMS is particularly useful is the ability to differentiate and then separately quantify parent and de-amidated species of peptides and/or proteins. Deamidation leads to only 0.985 Da difference in mass and even smaller shift in m/z due multiply charged ions common for peptides and proteins thus making it impossible to resolve using QQQ instruments and requiring lengthy chromatographic separations [17].
Another important application of qHRMS is for the mass isotopomer distribution studies (MIDA) that can be applied to study various metabolic pathways through flux analysis [18, 19]. For this application, ability to correctly and unambiguously differentiate between different isotopomers and simultaneously obtain quantitative information is critical. Utilization of HRMS for MIDA would lead to improved data quality as it would no longer require corrections for spill-over from neighboring isotopomers into the isotopomer of interest (while still requiring correction for natural heavy isotope abundance). This is particularly true for the M+1 isotopomer of a molecule where contribution from the M0 isotopomer is substantial when utilizing QQQ instruments operating at unit resolution [20].
Most recently, advances in both instrumentation and software have enabled absolute quantification of large intact proteins (e.g., antibodies) in biological matrices from samples collected from in vivo studies [21]. This innovative approach circumvents the need to rely on peptide(s) generated using trypsin or another protease for quantification of the abundance of large proteins by LC–MS/MS thus reducing the number of steps required for sample processing. It also enables a more comprehensive characterization of the post-translational modifications and/or biotransformation of the protein of interest without the need to obtain near complete sequence coverage from various tryptic digest fragments, which is often technically challenging to obtain and requires significant method optimization. However, the sensitivity reported in this study is still quite low (LLOQ = 1 µg/mL) and the range is quite narrow (1–30 µg/mL), which is of limited utility for detailed pharmacokinetic studies. Therefore, this approach requires further optimization to enable its more broad application in the field of bioanalysis.
Perspective on Regulated Bioanalysis
While QQQ instruments still dominate the field of regulated bioanalysis, qHRMS in full scan mode offers a novel challenge as well as opportunity for regulated bioanalysis. In case of HRMS, data can be re-interrogated after acquisition and disparate results obtained using different processing algorithms. Significant validation and documentation of processing methods would be required for applying qHRMS in full scan mode to regulated studies. In particular, the mass selection window used to generate the XIC is a critical parameter that can affect signal/noise ratio and thus assay performance.
For regulated assays, mass selection window has to be defined during assay development and confirmed during assay validation. Additionally, the question of validity of data, from regulatory point of view, obtained using criteria applied on post-hoc basis require further consideration. Moreover, the ability to mine full scan data in the case of clinical samples requires that appropriate informed consent is obtained prior to such analyses and is allowed by the study protocol.
MS/MSHR offers an alternative approach for applying qHRMS to regulated bioanalysis. In this workflow, one can define the targeted analytes a priori just as one would do on a QQQ. In this case, one can then capitalize only on the high resolving power of HRMS instrument employed to ensure superior specificity. We look forward to updated agency guidance on application of qHRMS to regulated studies.
Conclusion
In summary, qHRMS is becoming more widely applied to a variety of workflows ranging from discovery applications in the proteomic and metabolomics space and more regulated bioanalysis. Future users are encouraged to carefully consider the bioanalytical problem at hand and then determine whether their particular challenge can be addressed by the application of qHRMS or whether more traditional QQQ instrumentation is still sufficient.
References
- Lane AN, Fan TW, Xie Z et al. Isotopomer analysis of lipid biosynthesis by high resolution mass spectrometry and NMR. Anal Chim Acta. 651(2), 201–208 (2009).
- Yin Y, Han G, Zhou J et al. Precise quantification of mixtures of bispecific IgG produced in single host cells by liquid chromatography-orbitrap high-resolution mass spectrometry. MAbs doi:10.1080/19420862.2016.1232217 (2016).
- Frey AJ, Feldman DR, Trefely S et al. LC-quadrupole/orbitrap high-resolution mass spectrometry enables stable isotope-resolved simultaneous quantification and (1)(3)C-isotopic labeling of acyl-coenzyme A thioesters. Anal Bioanal Chem. 408(13), 3651–3658 (2016).
- Oppermann FS, Klammert M, Bobe C et al. Comparison of SILAC and mTRAQ quantification for phosphoproteomics on a quadrupole orbitrap mass spectrometer. J Proteome Res. 12(9), 4089–4100 (2013).
- Ranasinghe A, Ramanathan R, Jemal M et al. Integrated quantitative and qualitative workflow for in vivo bioanalytical support in drug discovery using hybrid Q-TOF-MS. Bioanalysis. 4(5), 511–528 (2012).
- Williamson LN, Bartlett MG. Quantitative liquid chromatography/time-of-flight mass spectrometry. Biomed Chromatogr. 21(6), 567–576 (2007).
- Kaufmann A, Butcher P, Maden K et al. Comprehensive comparison of liquid chromatography selectivity as provided by two types of liquid chromatography detectors (high resolution mass spectrometry and tandem mass spectrometry): “Where is the crossover point?” Anal Chim Acta. 673(1), 60–72 (2010).
- Fung EN, Jemal M, Aubry AF. High-resolution MS in regulated bioanalysis: where are we now and where do we go from here? Bioanlysis. 5(10), 1277–1284 (2013).
- Cazares LH, Ward MD, Brueggemann EE et al. Development of a liquid chromatography high resolution mass spectrometry method for the quantitation of viral envelope glycoprotein in Ebola virus-like particle vaccine preparations. Clin Proteomics. 13(1), 18 (2016).
- Gertsman I, Gangoiti JA, Barshop BA. Validation of a dual LC-HRMS platform for clinical metabolic diagnosis in serum, bridging quantitative analysis and untargeted metabolomics. Metabolomics. 10(2), 312–323 (2014).
- Glauser G, Veyrat N, Rochat B et al. Ultra-high pressure liquid chromatography-mass spectrometry for plant metabolomics: a systematic comparison of high-resolution quadrupole-time-of-flight and single stage orbitrap mass spectrometers. J Chromatogr A. 1292, 151–159 (2013).
- Kueger S, Steinhauser D, Willmitzer L, Giavalisco P. High-resolution plant metabolomics: from mass spectral features to metabolites and from whole-cell analysis to subcellular metabolite distributions. Plant J. 70(1), 39–50 (2012).
- Tong L, Zhou XY, Jylha A et al. Quantitation of 47 human tear proteins using high resolution multiple reaction monitoring (HR-MRM) based-mass spectrometry. J Proteomics. 115, 36–48 (2015).
- Lanshoeft C, Wolf T, Heudi O et al. The use of generic surrogate peptides for the quantitative analysis of human immunoglobulin G1 in pre-clinical species with high-resolution mass spectrometry. Anal Bioanal Chem. 408(6), 1687–1699 (2016).
- Esposito S, Bracacel E, Nibbio M et al. Use of ‘dilute-and-shoot’ liquid chromatography-high resolution mass spectrometry in preclinical research: application to a DMPK study of perhexiline in mouse plasma. J Pharm Biomed Anal. 118, 70–80 (2016).
- Paiva A, Shou WZ. Recent developments in software tools for high-throughput in vitro ADME support with high-resolution MS. Bioanalysis. 8(16), 1723–1733 (2016).
- Mehl JT, Sleczka BG, Ciccimaro EF et al. Quantification of in vivo site-specific Asp isomerization and Asn deamidation of mAbs in animal serum using IP-LC-MS. Bioanalysis doi: 10.4155/bio-2016-0035 (2016) (Epub ahead of print).
- Nilsson R, Jain M. Simultaneous tracing of carbon and nitrogen isotopes in human cells. Mol Biosyst. 12(6), 1929–1937 (2016).
- Kamphorst JJ, Fan J, Lu W et al. Liquid chromatography-high resolution mass spectrometry analysis of fatty acid metabolism. Anal Chem. 83(23), 9114–9122 (2011).
- Previs SF, Herath K, Castro-Perez J et al. Effect of Error Propagation in Stable Isotope Tracer Studies: An Approach for Estimating Impact on Apparent Biochemical Flux. Methods Enzymol. 561, 331–358 (2015).
- Jian W, Kang L, Burton L, Weng N et al. A workflow for absolute quantitation of large therapeutic proteins in biological samples at intact level using LC-HRMS. Bioanalysis. 8(16), 1679–1691 (2016).
ABOUT THE AUTHORS
Anton Rosenbaum currently a leads the mass spectrometry group within DMPK organization at MedImmune focusing on quantitative bioanalysis of biotherapeutics and identification and quantification of clinically relevant biomarkers. Prior to joining MedImmune, Anton was Senior Scientist at Pfizer Inc. where he led metabolomic research in non-alcoholic steatohepatitis. Prior to joining Pfizer, Anton did his postdoctoral training at Genentech Inc. where he championed application of mass isotopomer distribution analysis for the understanding metabolic pathways important in cancer. Anton obtained his PhD in Biochemistry and Structural Biology from the Weill Graduate School of Cornell University where his research focused on chemical biology dissection of intracellular cholesterol homeostasis.
Yue Huang has recently joined the mass spectrometry group within DMPK department at MedImmune as Scientist I. Yue is developing and validating methods for the quantification of biotheraputics in complex matrices utilizing LC-MS. Before joining MedImmune, Yue was a Scientist at Deurion LLC, developing novel ambient pressure ionization source for mass spectrometers. Prior to joining Deurion, Yue was Scientist I at Genzyme, focusing on protein degradation characterization and quantification with UPLC-MS/MS. Yue obtained her PhD from the Department of Chemistry from the University of Washington in Seattle. Her research focused on the mechanism and applications of SAWN (Surface Acoustic Wave Nebulization) as a novel ionization source for mass spectrometry.
MedImmune LLC, 319 N.
Bernardo Avenue, Mountain View,
CA 94043, USA
Contact info: [email protected], [email protected]