Current thinking on quantification of protein therapeutic pharmacokinetics and protein biomarkers in clinical studies
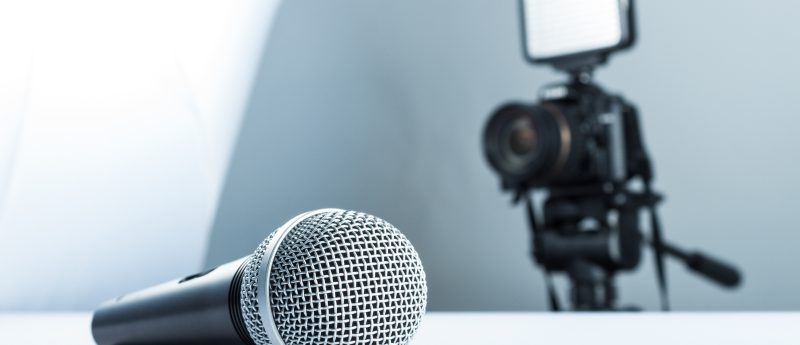
Kun Peng, PhD
Genentech, Inc., BioAnalytical Sciences, CA, USA
Email: [email protected]
Assessment of pharmacokinetics (PK) and biomarkers is an integral part of drug development. PK is usually an important endpoint in clinical studies, and PK results from both nonclinical and clinical studies are used for safety margin calculation, dose projection from animal to human and dose selection. Biomarkers have a variety of intended applications during different phases of drug development and together with PK data are key to establishing the PK/ pharmacodynamics (PD) relationship. Biomarkers may also be used as a diagnostic for patient selection or enrichment, a prognostic to determine outcomes independent of treatment, or as markers for PD and safety monitoring purposes. It is therefore essential to use bioanalytical methods of high quality to produce reliable PK and biomarker data to enable decision-making.
Quantification of protein therapeutics
Ligand binding assays (LBAs) are often considered as the method of choice for protein therapeutic PK measurements. Enzyme-linked immunosorbant assay (ELISA) is historically the most commonly used LBA format and has well-known technical and operational advantages and limitations. However, in recent years, choices of assay platforms for LBA development have become more diversified, which is largely credited to the advancement of new technologies, from electro-chemiluminescence platforms to a new generation of platforms including Gyrolab™ [1], Erenna® (Singulex) [2], Simoa (Quanterix) [3], and Immuno-PCR [4]. Compared with traditional immunoassay platforms, more recent technologies have clear technical advantages with the potential to address the bioanalytical challenges faced during biopharmaceutical drug development, such as high assay sensitivity requirement, increased throughput, reduced sample volume requirement and minimized matrix interference [5].
In addition to the immunoassay platforms mentioned above, affinity capture liquid chromatography tandem mass spectrometry (LC–MS/MS) methods have also been explored as an alternative platform for PK quantitation [6–8]. LC–MS/MS combines ligand-binding analyte enrichment with LC–MS detection and has demonstrated performance comparable to LBA. For example, LC–MS/MS has been successfully used to develop a generic plug-and-play assay for quantification of human IgG therapeutics in preclinical species [9]. In addition, LC–MS/MS is also a powerful tool to characterize drug-to-antibody ratio distributions for antibody–drug conjugate [10] and has been applied as an orthogonal approach to characterize and verify LBA data [11].
With the advancement of protein engineering technologies, the variety of engineered protein therapeutics is increasing and will continue to do so in the foreseeable future. These highly functional, customizable molecules have potential clinical benefits that traditional protein therapeutic formats could not offer. The increasing number of new modalities and alternative scaffolds pose new challenges for bioanalytical methods development [12]. The choices of PK quantification format will continue to evolve as we look to address new challenges. It will require significant collaborative efforts between biopharmaceutical, biotechnology and instrument companies to continue advancing bioanalytical platforms.
Quantification of protein biomarkers
Biomarkers are used widely with diverse applications during drug development. Therefore, a fit-for-purpose approach should be implemented to identify the best suited strategy for protein biomarker method evaluation (qualification versus validation). The recent draft FDA guidance lists fundamental parameters to be evaluated during assay validation [13,14]. This is the first time that the FDA has formally discussed the fit-for-purpose approach in the context of bioanalytical methods for biomarkers. While PK and biomarker assay validations share some similar evaluation parameters, there are distinct differences between the two that should be considered when evaluating biomarker assays.
One fundamental difference between PK and protein biomarker quantification is the source of the assay calibrator. In PK assays, the calibrator material is the well characterized and defined therapeutic, which is also the targeted analyte for quantification. In biomarker assays however, the analyte is an endogenous protein and the calibrator is commonly a partial or full-length recombinant protein produced in E. coli or mammalian cells. This material may or may not be representative of the endogenous protein and may possess intrinsic qualities that differ from the endogenous analyte, such as post-translational modifications, which may result in assay performance differences between the recombinant versus endogenous analytes. In this situation, the assay could demonstrate good performance using recombinant material during assay development and qualification, but may fall short during actual sample analysis. The comparison of recombinant and endogenous analyte materials should therefore be conducted early on during assay development.
While matrix interference is one of the key parameters assessed in a PK assay validation, it is particularly challenging for biomarker assays. Biomarker assays often have relatively higher sensitivity requirements, in the low pg/mL to fg/mL range in some cases. For PK assays one effective strategy to eliminate matrix interference is to prepare samples in high dilutions. However, this may not be feasible for biomarker assays because sample dilution needs to be kept low in order to achieve desired sensitivity. Hence, biomarker assays may require higher matrix tolerance compared to PK assays. When endogenous analyte levels are sufficiently high, experiments such as parallelism evaluation should be considered to demonstrate assay specificity and confirm minimally required dilution.
Commercially available biomarker kits provide a convenient and seemingly simple solution. Users are advised to carefully understand the method’s limitations and assess their suitability for a specific need [15,16]. Due to use of different assay reagents and criteria to define assay quality, it is not uncommon to find literature reporting the levels of the same analyte being very different, sometimes differing from hundred- to thousand-folds. Without a solid understanding of the methods used for data generation, it may lead to misinterpretation of the data and result in incorrect conclusions. If the commercial assay is intended for long-term use, one should also have a plan in place to mitigate lot-to-lot variations. Because the drug development process can span over years, it is not always feasible to use an assay from the same lot to support the entire development process. This is particularly challenging when new lots of key assay reagents, calibrator materials and capture and/or detecting reagents are incorporated.
Biomarker evaluation usually involves multiple analytes; therefore, platforms using reduced sample volume and/or with multiplexing capability remain attractive tools. For example, Simple PlexTM (Protein Simple) is an automated microfluidic platform that allows rapid and sensitive detection of multiple analytes using individual micro-channels for each analyte to eliminate cross-reactivity between the analytes [17]. LC–MS/MS is another platform that has improved sensitivity and multiplexing capacity for evaluation of complex panels of disease biomarkers [18,19].
Summary
Quantification of PK and biomarkers is an essential part of drug development. PK and biomarker readouts are largely determined by assay quality and format. Assay quality can be impacted by many factors, such as availability and quality of critical assay reagents, as well as limited assay development timelines. Often, the best assay strategy would be to provide the best performing assay using the available reagents within the required timeframe. It is therefore vital for bioanalytical scientists to understand the capabilities as well as limitations of an assay, and to communicate the relevant information to key stakeholders of the data so that these factors are taken into consideration when interpreting these data and making data-driven decisions.
Outsourcing bioanalytical work is a business model that many companies have adopted. It takes strong partnerships and effective communication to ensure that data of desirable quality are delivered in a timely manner. The emerging new technologies demonstrate clear analytical advantages; however, implementation of these technologies in contract laboratories can be challenging. The staff at contract laboratories requires additional training and experience to gain proficiency with the new platforms; successful implementation requires joint effort and extra attention from both sponsor and collaborating parties.
References
- Mora JR, Obenauer-Kutner L, Vimal Patel V. Application of the Gyrolab™ platform to ligand-binding assays: a user’s perspective. Bioanalysis 2(10), 1711–1715 (2010).
- Todd J, Freese B, Lu A et al. Ultrasensitive flow-based immunoassays using single-molecule counting. Clin. Chem. 53(11), 1990–1995 (2007).
- Rissin DM, Kan CW, Campbell TG et al. Single-molecule enzyme-linked immunosorbent assay detects serum proteins at subfemtomolar concentrations. Nat. Biotechnol. 28(6), 595–599 (2010).
- Ryazantsev DY, Voronina DV, Zavriev SK. Immuno-PCR: achievements and perspectives. Biochemistry (Mosc). 81(13), 1754–1770 (2016).
- Fischer SK, Joyce A, Spengler M et al. Emerging technologies to increase ligand binding assay sensitivity. AAPS J. 17(1), 93–101 (2015).
- Furlong MT, Ouyang Z, Wu S et al. A universal surrogate peptide to enable LC-MS/MS bioanalysis of a diversity of human monoclonal antibody and human Fc-fusion protein drug candidates in pre-clinical animal studies. Biomed. Chromatogr. 26(8), 1024–1032 (2012).
- Li H, Ortiz R, Tran L et al. General LC-MS/MS method approach to quantify therapeutic monoclonal antibodies using a common whole antibody internal standard with application to preclinical studies. Anal. Chem. 84(3), 1267–1273 (2012).
- Zhang YJ, Olah TV, Zeng J. The integration of ligand binding and LC-MS-based assays into bioanalytical strategies for protein analysis. Bioanalysis 6(13), 1827–1841 (2014).
- Kaur S, Liu L, Cortes DF et al. Validation of a biotherapeutic immunoaffinity-LC-MS/MS assay in monkey serum: ‘plug-and-play’ across seven molecules. Bioanalysis 8(15), 1565–1577 (2016).
- Kaur S, Xu K, Saad OM, Dere RC, Carrasco-Triguero M. Bioanalytical assay strategies for the development of antibody-drug conjugate biotherapeutics. Bioanalysis 5(2), 201–226 (2013).
- Peng K, Xu K, Liu L et al. Critical role of bioanalytical strategies in investigation of clinical PK observations, a Phase I case study. MAbs. 6(6), 1500–1508 (2014).
- Nuñez-Prado N, Compte M, Harwood S et al. The coming of age of engineered multivalent antibodies. Drug Discov. Today 20(5), 588–594 (2015).
- Lowes S, Ackermann BL. AAPS and US FDA Crystal City VI workshop on bioanalytical method validation for biomarkers. Bioanalysis 8(3), 163–167 (2016).
- Booth B, Arnold ME, DeSilva B et al. Workshop report: Crystal City V–quantitative bioanalytical method validation and implementation: the 2013 revised FDA guidance. AAPS J. 17(2), 277–288 (2015).
- Khan MU, Bowsher RR, Cameron M et al. Recommendations for adaptation and validation of commercial kits for biomarker quantification in drug development. Bioanalysis 7(2), 229–242 (2015).
- Fischer SK, Carrasco-Triguero M, Hong K et al. Commercial biomarker assays: friend and foe. Bioanalysis 8(22), 2351–2362 (2016).
- Aldo P, Marusov G, Svancara D, David J, Mor G. Simple Plex(™) : A novel multi-analyte, automated microfluidic immunoassay platform for the detection of human and mouse cytokines and chemokines. Am. J. Reprod. Immunol. 75(6), 678–693 (2016).
- Chen YT, Chen HW, Wu CF et al. Development of a multiplexed liquid chromatography multiple-reaction-monitoring mass spectrometry (LC-MRM/MS) method for evaluation of salivary proteins as oral cancer biomarkers. Mol. Cell. Proteomics 16(5), 799–811 (2017).
- Sangaraju D, Shahidi-Latham SK, Burgess BL, Dean B, Ding X. A multi-matrix HILIC-MS/MS method for the quantitation of endogenous small molecule neurological biomarker N-acetyl aspartic acid (NAA). J. Pharm. Biomed. Anal. 140, 11–19 (2017).
Financial Disclosure/Acknowledgment
The author is an employee of Genentech, Inc. and a stockholder of the Roche Group.
Our expert opinion collection provides you with in-depth articles written by authors from across the field of bioanalysis. Our expert opinions are perfect for those wanting a comprehensive, written review of a topic or looking for perspective pieces from our regular contributors.
See an article that catches your eye? Read any of our articles below for free.