Overview of clinical immunogenicity: whys and wherefores
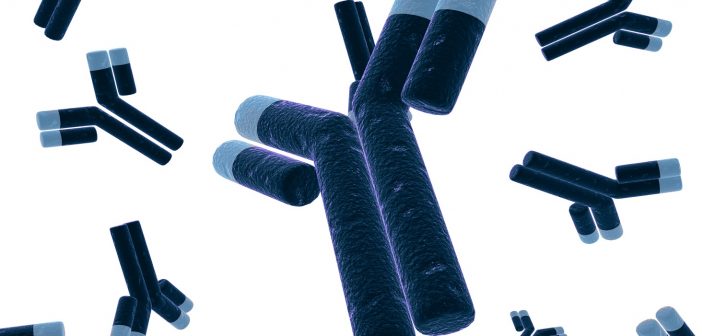
Eric Wakshull, PhD
Principal Scientist
Bioanalytical Sciences
Genentech
South San Francisco, CA 94080
Summary: This overview describes in brief the nature of the immune response to protein therapeutics, their clinical relevance, methodology for measuring it and approaches to interpreting the data.
The immunogenicity of therapeutic proteins is the result of the immune system doing its job —generating a response to a ‘foreign’ protein as a defensive mechanism to rapidly clear and/or neutralize the offending protein. From the perspective of patients, doctors and the pharmaceutical industry, this is an ‘unwanted’ immune response, as opposed to the desirable immune response elicited by an effective vaccine or by a pathogen (both of these topics are out of scope for this article), and is typically manifested by the production of host antibodies directed against the protein therapeutic termed anti-drug antibodies (ADAs). This is what the use of ‘humanized’ or ‘fully human’ proteins are designed to avoid, what many bioanalytical scientists spend time and resources devising ways to measure and characterize, and what health authorities around the world scrutinize carefully in drug approval applications.
With our current (albeit admittedly incomplete) understanding of how the immune system works, and in particular how nonself proteins are recognized and responded to by the production of antibodies to protein therapeutics, and our impressive abilities to engineer in or out virtually any structural feature desired in a protein, immunogenicity yet remains an ongoing concern for protein therapeutics. Potential reasons for this in the face of protein therapeutics engineered to be as native as possible will only be touched on briefly below. Rather, the focus of this short overview is to describe why measuring this immunogenic response is important, how we measure it and how we interpret the data derived from these measures.
Clinical relevance
Assessment of immunogenicity is a regulatory requirement and is considered part of the safety evaluation that all protein therapeutics undergo. It is generally believed, with considerable data at this point to back that belief, that all protein therapeutics are immunogenic to some degree. The most abundant class of approved protein therapeutics are monoclonal antibodies (mAbs), with most of the rest being growth factors, cytokines, or replacement enzymes [1].
For the vast majority of protein therapeutic ADA responses are relatively benign with little or no safety or efficacy impact. This is understandable for mAbs since they are generally constructed from constant domain consensus germline sequences with their complementarity-determining regions (CDR), like those of natural antibodies, being unique (either murine hybridoma-derived —‘humanized’, or human immunoglobulin derived from phage libraries, mice engineered with human immunoglobulin (Ig) genes, or cloned human B cells —termed ‘fully human’. Engineering mAbs in this way is thought to minimize immunogenic responses [2], and when appropriately characterized by epitope mapping ADAs against these mAbs are, like their endogenous anti-antibody counterparts [3,4], typically directed at the CDR [2,5]. Thus, mAb therapeutics are most likely to be removed from circulation as an immune complex with ADAs and/or neutralized by anti-idiotypic ADAs, without serious adverse events.
In such cases one can sometimes measure a loss of drug efficacy related to induction of ADAs (for example, [6–8]), but no safety events per se. While not strictly an ‘adverse safety event’ for life-saving therapeutics, ADAs that enhance drug clearance or neutralize drug activity are serious. In fact, a ‘call to arms’ occurred shortly after a report on the catastrophic effects of anti-recombinant erythropoietin (rEPO) ADA in which ADAs against the rEPO cross-reacted to endogenous EPO, the sole growth factor producing red blood cells, neutralizing its activity and inducing a pure red cell aplasia [9]. To mitigate these effects, various tolerance induction regimens have been evaluated and in some cases implemented [10].
ADAs can have clinically meaningful impact, and indeed some investigators have applied treatment algorithms that take induction of ADAs into account [11]. However, other growth factors inducing ADAs appear to be unimpaired in their therapeutic responses despite a large percentage of patients having measurable ADAs (>30%; e.g., insulin [12]; growth hormone [13]). The reasons that protein therapeutics induce an immune response, even when they are of identical sequence and activity to native proteins, can be related to host factors (patients with growth factor or enzyme deficiencies may have no immune tolerance to the protein) and product factors (e.g., protein aggregates or post-translational modifications [14–16]). Despite the clear associations of these factors with immunogenic responses, much is not understood from a mechanistic point of view.
Assay methodology
Assays for immunogenicity, measuring ADA against a protein therapeutic, are basically immunoassays that measure the ability of antibodies in a patient serum sample to bind to (and for neutralizing assays, neutralize) the drug. There are a number of variations on this theme, and multiple different technologies have been employed.
For non-mAb therapeutics a standard sandwich immunoassay is often used wherein the drug is immobilized either passively or via a linker such as biotin-drug to a streptavidin-coated microtiter plate. Plates are then incubated with serum samples, washed to remove nonbound proteins, and ADAs bound to the immobilized drug are detected. For example, using an anti-human Ig antibody from a nonhuman species that is conjugated to an enzymatic, fluorescent or electrochemiluminescent tag.
For humanized or fully human mAb therapeutics using an anti-human Ig detector would detect the immobilized drug along with the ADA, and is therefore not an effective format. Instead what is typically done is to use a bridging immunoassay format in which one batch of drug is conjugated to a biotin molecule (as noted above) and a separate batch of drug to a detector tag. These are then mixed together with a sample such that the ADA can form a bridge (thus, ‘bridging assay’) between the differently labeled drug — the biotin-drug is used to immobilize the biotin-drug/ADA/tag-drug complex to a solid surface, and the amount of ADA captured is measured by the detector signal. There are a number of variations on these two formats, and the curious reader can avail themselves of more comprehensive reviews [17–19].
There are a number of additional factors that need to be considered in developing and implementing immunogenicity assays. First and foremost is the decision threshold, also known as the ‘assay cutpoint’. This is the assay signal level that must be achieved to call a sample positive for the presence of ADA. There are no ADA ‘reference standards’ as there is for pharmacokinetic assays (i.e., the drug itself) because each patient’s immune response is both unique to them as well as evolving over time (isotype, affinity, binding epitope). Additionally, each patient’s sample biology and therefore background signal (i.e., before drug exposure) is different and can also change over time irrespective of the presence of ADA. Therefore, what is done is to calculate the mean value of the population background (typically 50–100 individual samples), and a cutpoint value is set at the 95th percentile of the population, by which a 5% false positive rate is achieved. This provides some assurance that low level ADA can be detected.
To refine this value by removal these potential false positive samples, a confirmatory assay is then used to assess the specificity of the putative positive signals — this is typically done by adding excess unlabeled drug to the aforementioned reaction mixture to inhibit binding events that are specific to the drug. Signals that are decreased by a specified amount (similar to the first cutpoint determining procedure described above, but using a 1% false positive rate) are considered to be confirmed positive. These two assays, screen and confirm, used in a tiered testing strategy, form the basic approach to evaluating the presence of ADAs in patients. Further characterization assays (titer, neutralizing; occasionally epitope or domain mapping) are then used as appropriate to better understand the nature of the immune response to the drug.
Another two factors require some mention. The first is drug tolerance. For many protein therapeutics, concentrations of drug in samples taken for immunogenicity assessment can be quite high. As in the confirmatory assay just described, this ‘unlabeled’ drug in the sample can inhibit ADAs from binding to the labeled drug, and if sufficiently high in concentration can produce a false negative result. Various strategies have been devised to enhance assay drug tolerance, the most common being the use of acid dissociation to disrupt drug/ADA complexes, then either with or without drug removal/blockage, pH neutralization followed by the addition of the labeled drugs to detect the now unbound ADA. There are a number of articles describing these and other methods [20–23], but their ability to enhance the detection of clinically meaningful ADA is not clear [24,25]. These and other considerations has led to the recent proposal to use the standard risked-based assessment [26] to modify if and when immunogenicity should be assessed [27].
The second factor is interference in immunogenicity assays by target. This can take the form of both positive interference (target acts like an ADA and generates assay signal above the cutpoint) leading to a false positive result ([28,29]; Wakshull, personal observation), or negative interference (target binds to drug and interferes with ADA that would otherwise bind at or near the target binding site; for example, ADA that might be neutralizing), leading to a false negative result. Both of these possible interferences should be assessed during assay development. A recent publication addresses these interferences and approaches to mitigate them [30].
Interpretation of clinical immunogenicity
ADA data in and of itself has minimal interpretative value in terms of evaluation of patient status. The most important use of ADA data is to assess their impact on relevant clinical endpoints. Typically, pharmacokinetic, pharmacodynamics, safety, and efficacy endpoints are chosen as being the most clinically meaningful that ADA might impact. Analysis is most frequently done simply by considering ADA-positive and ADA-negative patients as sub-groups, and their values compared. It is this kind of analysis that has allowed us to better understand the clinical relevance of ADA, as described earlier. But it can get fairly sophisticated depending on the amount of data available and can include parameters such as a) transient or persistent ADA, b) relative abundance (i.e., titer), c) time of ADA onset, d) neutralizing capacity, etc. [31]. In many cases, the incidence of immunogenicity (% of patients ADA positive) is too small to allow statistical analysis of an impact on clinical endpoints even in large pivotal trials, and a quick survey of drug labels shows many that make statements about trends or simply state that the impact of ADA is unclear. This is considered a good outcome, although it may mask the potential negative impact that can occur with individual patients. Sometimes a clearer picture emerges as additional data becomes available in the post-marketing environment. The way in which the analysis is done is specific to the drug, the patient population, the study design and of course the available data.
In this overview I have explained the nature of immunogenic response to protein therapeutics as a natural outcome of the normal function of the host immune system. There is ample literature demonstrating the clinical impact and importance of assessing this response and I have summarized both the methods used to measure ADAs and approaches to interpret their clinical relevance. There exists far more extensive literature than cited here, as well as guidance documents from regulatory authorities that present their recommendations and expectations for how and when sponsors will evaluate the immunogenicity of their products during nonclinical and clinical development (for the most recent regulatory health authority guidance’s see: [32,33].
References
- Lagasse HA, Alexaki A, Simhadri VL et al. Recent advances in (therapeutic protein) drug development. F1000Res. 113, 1–17 (2017).
- Harding FA, Stickler MM, Razo J, and DuBridge RB. The immunogenicity of humanized and fully human antibodies. 2(3), 256–265 (2010).
- Urbain J, Wikler M, Franssen JD, and Collignon C. Idiotypic regulation of the immune system by the induction of antibodies against anti-idiotypic antibodies. 74(11), 5126–5130 (1977).
- Hebert J, Bernier D, Boutin Y et al. Generation of anti-idiotypic and anti-anti-idiotypic monoclonal antibodies in the same fusion. Support of Jerne’s Network Theory. Immunol. 144, 4256–4261 (1990).
- Van Schouwenburg PA, de Stadt LA, de Jong RN et al. Adalimumab elicits a restricted anti-idiotypic antibody response in autoimmune patients resulting in functional neutralization. Rheum. Dis. 72, 104–109 (2013).
- Bartelds, GM, Krieckaert CL, Nurmohamed MT et al. Development of antidrug antibodies against adalimumab and association with disease activity and treatment failure during long-term follow-up. JAMA. 305(14), 1460–1468 (2011).
- Astemark J. FVIII inhibitors: pathogenesis and avoidance. Blood 125(13), 2045–2051 (2015).
- Kishnani PS, Dickson PI, Muldowney L et al. Immune response to enzyme replacement therapies in lysosomal storage diseases and the role of immune tolerance induction. Genet. and Meta. 117, 66–83 (2016).
- Casadevall N, Nataf J, Viron B et al. Pure red-cell aplasia and antierythropoietin antibodies in patients treated with recombinant erythropoietin. Engl. J. Med. 348(7), 469–475 (2002).
- Salazar-Fontana LI, Desai DD, Khan TA et al. Approaches to mitigate the unwanted immunogenicity of therapeutic proteins during drug development. J. 19(2), 377–385 (2017).
- Garces S, Antunes M, Benito-Garcia E et al. A preliminary algorithm introducing immunogenicity assessment in the management of patients with RA receiving tumour necrosis factor inhibitor therapies. Rheum. Dis. 73(6), 1938–1943 (2013).
- Fineberg SE, Kawabata TT, Kinco-Kent D et al. Immunological responses to exogenous insulin. Endocrine Rev. 28(6), 625–652 (2007).
- Romer T, Peter F, Saenger P et al. Efficacy and safety of a new ready-to-use recombinant human growth hormone solution. Endocrinol. Invest. 30, 578–589 (2007).
- Schellekens H. Factors influencing the immunogenicity of therapeutic proteins. Dial Transplant 20(S6), vi3–vi9 (2005).
- Ratanji KD, Derrick JP, Dearman RJ, and Kimber I. Immunogenicity of therapeutic proteins: influence of aggregation. Immunotoxicol. 11(2), 99–109 (2014).
- Haile LA, Puig M, Kelley-Baker L, Verthelyi D. Detection of innate immune response modulating impurities in therapeutic proteins. One 10(4), 1–17 (2015).
- Gelzleichter T and Wakshull E. Considerations on immunogenicity in the nonhuman primate in nonclinical drug development and safety assessment. Bluemel J, Konte S, Schenck E, and Weinbauer G (eds), Chapter 24, 457–467 (2015).
- Mikulskis A, Yeung D, Subarmanyam M, Amarvadi L. Solution ELISA as a platform of choice of development of robust, drug tolerant immunogenicity assays in support of drug development. Investig. Med. 365, 38–49 (2011).
- Menendez AT. Strategic selection and development of immunogenicity binding methods. Bioanalysis 4(12), 1491–1508 (2012).
- Patton A, Mullenix MC, Swanson SJ, Koren E. An acid dissociation bridging ELISA for detection of antibodies directed against therapeutic proteins in the presence of antigen. Investig. Med. 304, 189–195 (2005).
- Smith H, Butterfield A, and Sun D. Detection of antibodies against therapeutic proteins in the presence of residual therapeutic protein using a solid-phase extraction with acid dissociation (SPEAD) sample treatment prior to ELISA. Toxicol. Pharmacol. 49, 230–237 (2007).
- Bourdage JS, Cook CA, Farrington DL et al. An affinity capture elution (ACE) assay for detection of anti-drug antibody to monoclonal antibody therapeutics in the presence of high levels of drug. Investig. Med. 327, 10–17 (2007).
- Sickert D, Kroeger K, Zikler C et al. Improvement of drug tolerance in immunogenicity testing by acid treatment on biacore. Investig. Med. 334, 29–36 (2008).
- Van Schouwenburg, PA, Krieckaert CL, Rispens T et al. Long-term measurement of anti-adalimumab using pH-shift-anti-idiotype antigen binding test shows predictive value and transient antibody formation. Rheum. Dis. 72, 1680–1686 (2012).
- Bloem K, Hernandez-Breijo B, Marinez-Feito A, and Rispens T. Immunogenicity of therapeutic antibodies: monitoring antidrug antibodies in a clinical context. Drug Monit. In press 2017.
- Koren, E, Smith HW, Shores E et al. Recommendations on the risk-based strategies for detection and characterization of antibodies against biotechnology products. Investig. Med. 333, 1–9 (2008).
- Mytych DT, Hock MB, Kroenke M et al. A proposal to redefine clinical immunogenicity assessment. J. 19(3), 599–602 (2017).
- Zhong ZD, Dinnogen S, Hokom M et al. Identification and inhibition of drug target interference in immunogenicity assays. Investig. Med. 355, 21–28 (2010).
- Chen K, Page JG, Schwartz AM et al. False-positive immunogenicity responses are caused by CD20+ B cell membrane fragments in an anti-ofatumumab antibody bridging assay. Investig.Med. 394, 22–31 (2013).
- Zhong ZD, Clements-Egan A, Gorovits B et al. Drug target interference in immunogenicity assays: recommendations and mitigation strategies. J. 2017 accepted for publication.
- Shankar G, Arkin S, Cocea I et al. Assessment and reporting of the clinical immunogenicity of therapeutic proteins and peptides—harmonized terminology and tactical recommendations. J. 16(4), 658–673 (2014).
- FDA guidance for industry. Assay development and validation for immunogenicity testing of therapeutic protein products. 2016 draft guidance.
- EMA guideline on immunogenicity assessment of biotechnology-derived therapeutic proteins. 2017. EMEA/CHMP/BMWP/14327/2006 Rev.1.
Back to immunogenicity