Learn with Lin about therapeutic antibodies: part II − how it works
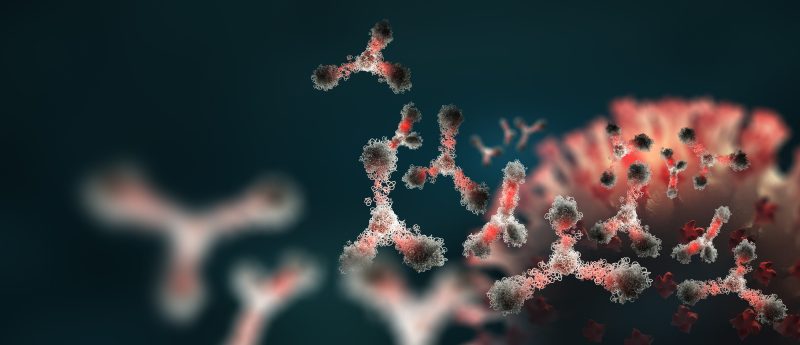
Lin Wang, Regeneron Pharmaceuticals (NY, USA)
“Hello everyone, this is Lin! I am back with a new ‘Learn with Lin’ series! Over the past 25 years, antibodies have revolutionized the pharmaceutical industry and patients’ lives. Between 1986 and 2021, there were approximately 126 commercially available antibodies approved globally. Prior to 2014, there were less than five approved each year. However, over the last 4 years, there were more than 14 antibody approvals [1]. Therapeutic antibodies now heavily dominate the biologics market and continue to present a significant industrial interest [2].The monoclonal antibody (mAb) therapeutics market is segmented into several categories including cancer, autoimmune diseases, infection, hematological diseases and others [3]. Their high specificity and affinity for targeted antigens are the critical reasons for their employment.”
In this instalment, we will discuss how antibodies protect us from a variety of diseases and focus on their applications in cancer and virus infection like COVID-19.
Actions of antibodies
In our body, antibodies defend us against countless harmful factors mainly through the following four ways: neutralization, opsonization, complement-dependent cytotoxicity (CDC), and antibody-dependent cellular cytotoxicity (ADCC).
Neutralization
Many pathogens cause diseases by binding to the surface molecules of the host cell, causing them to gain entry into the cell. Antibodies can bind to these pathogens and block them, or in other words, neutralize their infectivity, thus preventing them from entering the cells. Neutralized pathogens will be eliminated by phagocytosis eventually.
Opsonization
Opsonization is a process which enhances phagocytosis. Antibodies coat the surface of the pathogen by binding to specific antigenic determinates. Phagocytic cells have specific Fc receptors which can recognize the bound antibodies. Once the phagocytes find these antibodies, the pathogens are eliminated by phagocytosis. Here, antibodies act as opsins, which forms a bridge between the pathogen and the phagocyte.
CDC
When the antibody binds to the surface of an antigen, it can activate a reaction named the classical pathway of the complement system. The complement system consists of a number of small proteins. Once it is activated, the proteases in the system cleave specific proteins and initiate an amplifying cascade of further cleavages. These series of reactions will result in the stimulation of phagocytes, inflammation to attract additional phagocytes and the formation of the membrane attack complex (MAC), which creates pores that disrupt the cell membrane of target cells, leading to cell lysis.
ADCC
When an intracellular infection occurs or the invading pathogen is too large, for example, parasites, phagocytosis is not effective any more to remove these pathogens. In these two cases, our immune system develops another strategy. The infected cell displays a foreign antigen on their plasma membrane which antibodies will bind to. The Fc region of these bound antibodies are then recognized by leukocytes, like natural killer cells and eosinophils, through Fc receptors on the surface of these cells. This recognition will trigger the degranulation of these leukocytes and release the cytotoxic chemicals, resulting in the lysis of the pathogen or infected cells.
Role in COVID-19 pandemic
It is no exaggeration to say that the COVID-19 pandemic has changed the world. Our daily necessities have been greatly affected. The novel coronavirus, SARS-CoV-2, that caused this pandemic is an enveloped, positive-sense, single-stranded RNA virus [4]. It contains RNA inside and several important structural proteins on its surface (shown in Figure 1). One of the most notorious proteins is the spike protein.
Figure 1. Structure schematic of SARS-CoV-2.
The spike protein is responsible for allowing the virus to attach to the host cell membrane. It has a receptor binding domain (RBD), which recognizes a specific receptor called angiotensin converting enzyme receptor 2 (ACE2). The spike protein can be further divided into two functional subunits: S1 and S2. S1 binds to the host cell receptor and S2 mediates the fusion of the viral and cellular membranes.
How does the coronavirus infect cells [5,6]?
It starts with the spikes. When the virus particles encounter respiratory epithelium cells, the S1 subunit binds to receptor ACE2. Next, the virus can enter the host cell in two ways. One way is through an endosome – a lipid-surrounded bubble with the help from a host protease enzyme called cathepsin L. When the virus enters cells by this route, antiviral proteins can trap it. The other way is faster and efficiently uses another host protease enzyme called transmembrane protease, serine 2 (TMPRSS2), which is found in high amounts on the outside of respiratory cells. In this article, we will focus on the TMPRSS2 route.
After binding to ACE2, it induces conformational changes in the S1 subunit and exposes a specific cleavage site in the S2 subunit. TMPRSS2 helps the cut and further exposes a run of hydrophobic amino acids that rapidly buries itself in the closest membrane of the host cell. It also dissociates S1 from S2 and induces dramatic conformational changes in the S2 subunit. The extended spike will then fold back onto itself, pulling the membranes of the cell and virus together so they fuse. After fusion, the virus ejects its genome directly into the cell, where it uses the cell’s machinery to produce more virus particles.
Can therapeutic antibodies neutralize SARS-CoV-2?
From the above, every step of the virus infection process seems to be very precise and ingenious. So, is there any step in this that we can intervene? The answer is yes.
Several therapeutic antibodies were developed either from genetically humanized mice or convalescent humans to inhibit SARS-CoV-2 infection. For example, RBD-targeting antibodies will bind to the virus and form RBD/mAb complexes that inhibit binding of the RBD to ACE2, thereby preventing the attachment of SARS-CoV-2 to the host cells [7]. One thing that is worth mentioning is that RNA viruses are well known to accumulate mutations over time. Only one kind of highly potent neutralizing antibodies may not be sufficient to protect against the rapid generation of viral escape mutants. As demonstrated from one article published in Science, antibody cocktails targeting distinct and non-overlapping regions of the RBD of spike protein can have the potential value to minimize the mutational escape. This makes it much more difficult for the virus to mutate because it requires simultaneous mutations at more than one distinct genetic site [8].
Figure 2. SARS-CoV-2 infection process and antibodies’ role.
Cancer, Cancer, Cancer
Cancer, a word that can probably sends a shiver down the spine, is a disease caused by the uncontrolled growth of abnormal cells. Generations of men and women have been battling against this formless malady for 4000 years. However, at present, cancer remains as one of the leading causes of death in the world. The good news is that, in recent years, antibody-based therapy for cancer has achieved considerable success. Over 35 antibodies have been approved by the US FDA for cancer treatment so far [9]. Uncontrolled growth is the deadly core of cancer, but this also differentiates them from normal cells. The key to curing cancer is to find the suitable targets that are overexpressed, mutated, or selectively expressed by cancer cells compared with normal cells, and then use antibodies to action on them precisely [10]. The complex mechanisms of tumor cell killing by antibodies can be simplified into three words: block, flag and deliver.
Figure 3. Examples of antibodies’ actions in cancer treatment [11].
- Block
Antibodies can bind to certain growth factors, growth factors receptors, or immune checkpoints to inhibit necessary signaling. For example, an approved antibody targets a receptor on the outside of breast cells called human epidermal growth factor receptor 2 (HER2). HER2 receptors are like ears, receiving signals that stimulate the cell to grow and multiply. But these receptors are overexpressed on the surface of breast cancer cells. Thus, these cancer cells grow and spread faster due to picking up too many growth signals. The antibodies can bind to HER2 receptors, block them from receiving growth signals and slow down the growing of cancer cells [12]. Another example involves a growth factor called vascular endothelial growth factor (VEGF). It is a signaling protein that promotes the growth of new blood vessels. By binding to this factor, antibodies can prevent it from binding to its own receptor and inhibit endothelial cell proliferation and vessel formation, which tumors need to survive [13]. A third example is the interaction with immune checkpoints. Checkpoints are molecules on certain immune cells to help them identify normal cells and ‘foreign’ cells. However, cancer cells can find ways to use this function and disguise themselves from being attacked.
For instance, PD-1 (programmed death-1) is a checkpoint protein on T cells and acts as an ‘off switch’. When PD-1 attaches to its partner protein PD-L1 (programmed death-ligand 1), they send an ‘off’ signal to the T cells and prevent the immune system from destroying the cells. Some cancer cells have large amounts of PD-L1, which helps them hide from immune attacks. Another target checkpoint is called CTLA-4 (cytotoxic T lymphocyte-associated antigen-4), which also acts as a type of ‘off switch’. Antibodies targeting checkpoint proteins are called checkpoint inhibitors. They can bind to these checkpoint proteins to block them from attaching to their partner ligands. This prevents the ‘off’ signal from being sent, allowing the T cells to kill cancer cells [14].
- Flag
In our body, there are some cell surface markers defined as cluster of differentiation (CD). Each unique surface molecule is assigned a different number, which allows identification and characterization of cell phenotypes [15]. For example, CD20 and CD30 are proteins found on either B cells or T cells. They may be found in higher-than-normal amounts in patients with certain types of lymphomas and leukemias. Many antibody therapies were successfully developed targeting these two antigens. These antibodies can bind to these tumor markers via the variable region and flag these cancer cells. The Fc region will activate other immune responses, like CDC and ADCC, to get rid of the cancer cells.
Another promising approach is through the dual binding mechanism of bispecific antibodies (bsAbs), which can attach to two different antigens simultaneously. For example, some bispecific antibodies can bind to CD20 and CD3. CD20 is overexpressed on malignant B cells and CD3 is on T cells, functioning as a T-cell activator. Binding these two with one arm from antibodies attaching to CD20 and one arm to CD3 can redirect T cells to engage and eliminate malignant B cells [16].
- Deliver
For this mechanism, antibody conjugates are the leading actors. An antibody−drug conjugate (ADC) consists of a cytotoxin covalently linked to a mAb. In this way, it can be ensured that while the antibody finds its target, it can also carry toxic substances to kill cancer cells. They can perform the duty of chemotherapy and radiation but also spare normal cells due to their high specificity.
Summary
In this article, we have looked at the basic functions of antibodies and their roles in COVID-19 and cancer treatment. However, the mechanisms involved are actually very complex, especially for cancer therapies. Compared with the past, we have made great progress in medicine. But it is possible that the great mystery of life has only been pulled up by a tiny corner and the journey of exploration is far from over.
References
- Strickley RG, Lambert WJ. A review of formulations of commercially available antibodies. J. Pharm. Sci. 110(7), 2590−2608 (2021).
- Sifniotis V, Cruz E, Eroglu B, Kayser V. Current advancements in addressing key challenges of therapeutic antibody design, manufacture, and formulation. Antibodies, 8(2), 36 (2019).
- GlobeNewswire. Global monoclonal antibody therapeutics market will reach USD 218.97 billion by 2023: Zion Market Research. (2018): https://www.globenewswire.com/news-release/2018/04/10/1467446/0/en/Global-Monoclonal-Antibody-Therapeutics-Market-Will-Reach-USD-218-97-Billion-by-2023-Zion-Market-Research.html
- Schmidt N, Lareau CA, Keshishian H et al. The SARS-CoV-2 RNA–protein interactome in infected human cells. Nat. Microbiol. 6(3), 339−353 (2021).
- Jackson CB, Farzan M, Chen B, Choe H. Mechanisms of SARS-CoV-2 entry into cells. Nat. Rev. Mol. Cell Biol. 1−18 (2021).
- Scudellari M. How the coronavirus infects cells-and why Delta is so dangerous. Nature, 595(7869), 640−644 (2021).
- Yang Y, Du L. SARS-CoV-2 spike protein: a key target for eliciting persistent neutralizing antibodies. Signal Transduct. Target. Ther. 6(1), 95 (2021).
- Baum A, Fulton BO, Wloga E et al. Antibody cocktail to SARS-CoV-2 spike protein prevents rapid mutational escape seen with individual antibodies. Science, 369(6506), 1014−1018 (2020).
- Zahavi D, Weiner L. Monoclonal antibodies in cancer therapy. Antibodies, 9(3), 34 (2020).
- Scott AM, Wolchok JD, Old LJ. Antibody therapy of cancer. Nat. Rev. Cancer, 12(4), 278−287 (2012).
- Maloney DG. Anti-CD20 antibody therapy for B-cell lymphomas. N. Engl. J. Med. 366(21), 2008−2016 (2012).
- Gajria D, Chandarlapaty S. HER2-amplified breast cancer: mechanisms of trastuzumab resistance and novel targeted therapies. Expert Rev. Anticancer Ther. 11(2), 263−275 (2011).
- Ranieri G, Patruno R, Ruggieri E, Montemurro S, Valerio P, Ribatti D. Vascular endothelial growth factor (VEGF) as a target of bevacizumab in cancer: from the biology to the clinic. Curr. Med. Chem. 13(16), 1845−1857 (2006).
- National Cancer Institute. Immune checkpoint inhibitors. (2019): https://www.cancer.gov/about-cancer/treatment/types/immunotherapy/checkpoint-inhibitors
- Actor JK. Chapter 1 – A Functional Overview of the Immune System and Immune Components. In Introductory Immunology (Second Edition), Actor JK, Ed. Academic Press: 1−16 (2019).
- Pharmacy Times. Bispecific antibodies: the next generation of immunotherapy. (2020): https://www.pharmacytimes.com/view/bispecific-antibodies-the-next-generation-of-immunotherapy