Interview with Leroy Hood (Institute of Systems Biology) on receiving the National Medal of Science
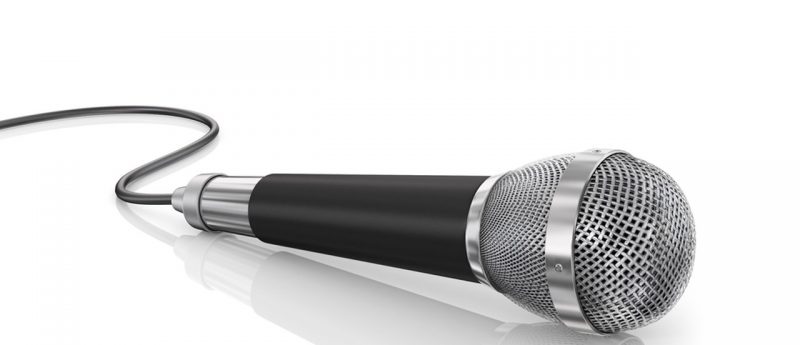
Leroy Hood received an MD from Johns Hopkins and a PhD from Caltech. He was a professor at Caltech for 22 years, at the University of Washington for 8 years and has been President and co-founder of the Institute for Systems Biology for the past 13 years. His interests include technology development, molecular immunology, genomics, systems biology, cancer, and neurodegeneration. He has published more than 700 papers, has 36 patents and has been involved in the start-up of 13 different companies. He was won numerous awards including the Lasker Award (1987), the Kyoto Prize (2002), the Russ Prize (2011) and most recently the Medal of Science (2011). He is a member of the National Academy of Sciences, the National Academy of Engineering and the Institute of Medicine. He is currently focused on taking a systems approach to disease.
How did it feel to be presented with the ‘National Medal of Science’ at the White House?
It was an unexpected and very exciting announcement. I found it very special in two ways. First I have been involved in a series of paradigm changes in biology some of which have been very skeptically received by the community and this award was a validation of these paradigm changes and their success over the 47 years of my career. Second, I was really delighted in the sense that it validated the many really terrific co-workers that I have had with me over the course of my career. I think the medal acknowledges those who did the work as well as my direction of the work.
Please can you tell us a bit about the work involved that led to you winning the award?
The award is for a lifetime of achievement so I would guess probably that there are three major accomplishments. One was the series of paradigm changes, which included bringing technology to biology, the human genome project, cross-disciplinary biology, systems biology and systems medicine. A second was the development of technologies and strategies for doing biology that have been characteristic of my laboratory throughout my career, namely machines that could sequence, synthesize and characterize both DNA and proteins. One of the major tools that we developed was the automated DNA sequencer and that enabled the human genome project (HGP) to be achieved. It was the development of this tool that actually got me involved in the very early years of the HGP in 1985 and I was an advocate from 1985 to 1990 when I would say that 90% of the biologists were extremely skeptical. The National Institutes of Health (NIH) was against the HGP. Importantly, it was the Department of Energy (DOE) that actually pioneered and pushed through the initial effort that ultimately led to the HGP. Finally, I think we had some beautiful biological discoveries in molecular immunology, in neurobiology and more recently in cancer biology. The award probably included a consideration of all three of these areas.
At what stage in your career did you first become involved in systems biology and what first attracted your attention to this area?
I was studying molecular immunology in the 1970s and 1980s and I came to the conclusion that the immune system was unbelievably complex. Looking at one gene and one protein at a time was just not going to work in unraveling its complexities. I began thinking about systems biology in the early 1980s but we lacked a list of human genes (and by inference proteins). The human genome project gave this list. We also lacked many critical tools like high speed DNA sequencing and DNA arrays. As these tools were developed, systems biology began to emerge in the middle of the 1990s. We founded the Institute for Systems Biology in 2000 — the first of its kind — and the exponential explosion of systems biology was initiated.
Please can you tell us a little about the novel concept of P4 medicine research?
P4 medicine is the convergence of three major forces in medicine today — systems medicine — the systems-biology approach to disease, big data and its powerful analytics and patient-activated social networks. In 10 years each patient will be surrounded by a virtual cloud of billions of data point from many different types of data (molecular, cellular, clinical, social, etc). P4 medicine will have the computational tools to reduce this enormous data dimensionality to simple hypotheses about optimizing wellness and minimizing disease for each individual. The fundamental concept of P4 medicine is that in disease one of your basic biological networks becomes disease-perturbed and as the disease progresses — additional networks become disease perturbed. This process leads to altered information expressed in the individual that given rise to disease and its progression. Insights into the dynamics of these disease-perturbed networks will provide fundamental insights into disease mechanisms and into new approaches to diagnosis and therapy. Thus P4 medicine is proactive, focuses on the individual patient, is committed to optimizing wellness and gathers enormous amounts of data for each individual. P4 medicine is about optimizing wellness and minimizing disease for each individual. My belief is that it is the patient-activated social network that will drive the acceptance of P4 medicine in the medical community — physicians and the medical community are quite conservative and waiting for their acceptance would delay this process significantly.
Regarding your recent collaboration with AB SCIEX in this area, what have been the main challenges associated with this partnership?
The main challenges associated with The AB SCIEX/ISB partnership are taking a marvelous new mass spectrometer and workflow, SWATH™ Acquisition, and developing the applications that will allow us to make global and comprehensive measurements. The essence of what SWATH does is to take a complex mixture of proteins, called the proteome, which can be from a single cell or an organ or even from a whole individual, convert them into enzymatically digested tryptic peptides and then digitalize peptide measurements so that we will be able to deconvolute and quantify each of the tryptic peptides and hence each of the corresponding proteins. It is a similar analysis to what Google does with its program to visualize the earth. It digitalizes a map of the earth and then you can focus on whatever region of the earth you would like to examine in whatever degree of resolution they provide with the digitization process. SWATH creates a similar digital record of each of the peptides in the complex proteome. A second challenge that ISB is committing to is to develop the computational proteomics software to convert the digital record of the peptides into identification and quantification of the corresponding proteins. This approach will give proteomics the power to make global measurement in precisely the manner that genomics has been able to do for some time. It is a major step forward in technology. It will enable powerful new approach to systems biology and systems medicine.
You have been involved in the founding of 13 biotechnology companies throughout your career: what are the problems one faces when setting up a new company in the field?
I think that there are three problems. First, you have to articulate a vision for the company that makes sense in business terms, what you are going to do and how you are going to make money for the investors. Second, you have to find the people that will back the company–the venture capitalists, the investment bankers, the industrial investors and/or the angel investors. Third, you need to find really good managers. My feeling about companies is it is really about the Chief Executive Officer (CEO) that will determine the success or failure of a company. A poor CEO can take a great idea and fail with it; a great CEO can take a poor idea and make it successful. Selecting the management for the company is really critical. I would say those are the three most important objectives that you have to meet when creating a new company that is going to be successful.
In your opinion, what have been the most important milestones during your time as a research scientist in the systems biology field?
I would say one important milestone was convincing the world that systems biology was real. When we started the Institute for Systems Biology in 2000, there was enormous skepticism about systems biology and the general feeling that it was just hype. This skeptical view has been dispelled by the many successes of systems biology. Indeed, my perceptions about the power of systems biology have been validated by a 2009 report by the National Academy of Sciences on the “new biology” which describes perfectly systems biology. This report says that the ability of systems biology to deal with biological complexity is the key to the future of the life sciences for diverse areas including medicine, agriculture, energy and the environment. Some of the authors of that report were among the skeptics 12 years ago when we started the institute — so there has been a fundamental acceptance of the importance of systems biology.
I would say the second milestone is systems biology has shown us clearly that we can take complex biological systems and begin to deconvolute and understand in enormous detail their inner workings. The pioneering work of Eric Davidson at Caltech in which he took a systems approach to understanding the first 3 days of sea urchin development is a classic example. This development can virtually be entirely explained by understanding the dynamics of their many biological networks. Indeed, Eric has used this detailed dynamical network map to explain most of the major observations made in the past 100 years of experimental sea urchin development. And this is but one milestone. Our own work on explaining prion-induced neurodegeneration in terms of the dynamics of disease-perturbed biological networks is yet another example. This is a new approach to understanding the complexities of biology.
Third, the development of the automated DNA sequencer was a real milestone — for it not only enabled the completion of the human genome project, but it foreshaddowed the high throughput genomics of today that is a centeral feature of the big data that systems biology requires. It led to a fundamental transformation in medicine as for each individual we have a complete genome sequence that will enable us over the next 10 years to begin to optimize wellness and minimize disease (see below).
Finally, I would say a fourth milestone has been the emergence of systems medicine and the demonstration that is already starting to transform the practice of healthcare — providing more powerful ways to identify disease genes, making blood a window into distinguishing health from disease, providing the means for stratifying disease (e.g. breast cancer) into its different subtypes so that there can be an proper impedance match against effective drugs and pioneering the realization that medicine must focus on each individual in the context of their own data. Systems medicine is leading to a healthcare that is more effective, cheaper and promotes innovation.
In the next 5–10 years, what particular element of systems biology do you see advancing the most and why?
I would say there are really two areas for advancement — technology and the analytical tools analytical tools that will let us handle enormous amounts of data and extract signal from noise. We are going to develop a series of technologies that will let us analyze complex biological systems more precisely, more globally and on smaller samples than we can now use. The SWATH MS-based proteomics approach and a 3rd generation DNA sequencer that will be able sequence a complete human in 15 minutes are examples. Another really important area is going to be the detailed analysis of single cells so we can essentially look and characterize the most fundamental quantized unit of information in living organisms — the cell. In a brain or in a liver cells may appear identical, but they are actually divided into different quantized populations specified by transcription factors. These quantized cell populations perform different functions and interact to execute complex functions. We can come to identify and come to understand how these differences quantized populations of cells actually facilitate biological functions.
The second advance will be to validate, mine, and integrate big data so that ultimately we can create models that are both predictive and in the case of medicine actionable. The modeling will require integrating across different scales of data — genetic, to molecular to cellular to organs to of tumors and even organs — assessing how they interact with their environment — to create multi-scale models in biology is one of the grand challenges of systems biology. I predict in the future that we will be able to take a tumor with its billions upon billions of data points and model it with incredible clarity and accuracy and make predictions about what is the best therapeutic approach and how you can reverse the effects of mutations that might be taking the tumor away from the optimal originally therapy.
What words of encouragement would you give young scientists who might be envisioning a career in systems biology science?
I would make three comments. First, across the 47 years of my career, now is the most exciting time ever in science. There are absolutely spectacular opportunities for creative and imaginative young scientists. Second, there are fascinating areas of science that can be transformed with a cross-disciplinary and systems approach. For example, nutrition is a key area of human health that is very immature. A young scientist asking the right kinds of questions could transform this field — and could be a pioneer in systems nutrition. There are also similar opportunities in the areas of agriculture, energy, the environment and global health. Finally, I think that a cross-disciplinary and systems training is going to be vital for young scientists that want to pioneer new field. You need a good background in chemistry, physics and statistics. I would advise you to take dual majors — biology and something orthogonal like computer science. I would argue that every 10 years you should be prepared to change careers and explore a new field — that will keep you always young. The big challenges that young scientists face today are obtaining a position and funding for research. The key is determined optimism — for I am convinced that both of these challenges will turn around — although they may do so through creating scientific institutions that have very different business strategies today. There are and will continue to be enormous opportunities in science for the young scientist.