High-resolution mass spectrometry: more than exact mass
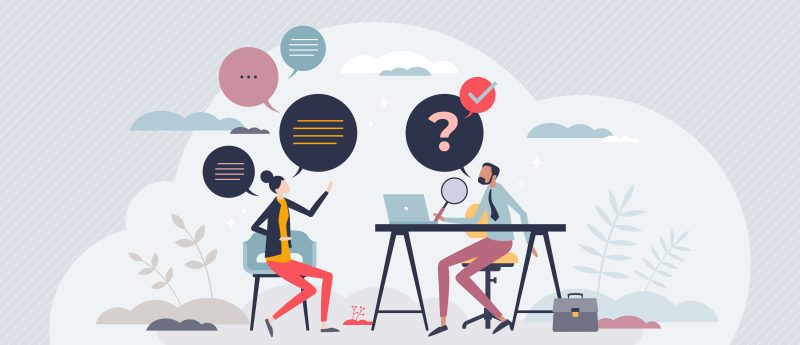
- High-resolution mass spectrometry: more than exact mass
- Why is measuring ‘exact mass’ with high-resolution mass spectrometry useful?
- Does the existence of high-resolution mass spectrometry preclude the use of ‘conventional’ mass spectrometry?
- How does high-resolution mass spectrometry work?
High-resolution mass spectrometry: more than exact mass
The applications of high-resolution mass spectrometry (HRMS) are a hotly debated topic in the field of bioanalysis. In the most basic terms, HRMS can measure the mass to charge ratio (m/z) to several decimal places. The ‘higher’ resolution means that, rather than nominal masses, ‘exact masses’ can be measured.
For example, take cysteine and benzamide. Both molecules have a nominal mass of 121. Conventional MS would not be able to distinguish the two. By calculating the exact mass of each compound and measuring your sample using HRMS, it becomes possible to distinguish the analytes:
- Cysteine (C3H7NO2S): (3 x 12.0000) + (7 x 1.0078) + (1 x 14.0031) + (2 x 15.9949) + (1 x 31.9721) = 121.0196
- Benzamide (C7H7NO): (7 x 12.0000) + (7 x 1.0078) + (1 x 14.0031) + (1 x 15.9949) = 121.0526
If a high-resolution mass spectrometer measures an m/z of 121.0525, it becomes possible to identify the analyte in question.
Why is measuring ‘exact mass’ with high-resolution mass spectrometry useful?
But why is being able to measure ‘exact mass’ useful? After all, technological developments of high performance triple quadrupole mass spectrometers facilitate mass resolution down to 0.1 Da [1]. It is also worth noting that measuring exact mass only affords the distinction between molecules with different formulas but the same nominal mass. HRMS alone cannot be used to distinguish isomers.
Nevertheless, non-high-resolution mass spectrometers, including ion trap linear quadrupole configurations, are generally capable of measuring to integer resolutions. Such MS techniques can be used to distinguish a compound with a nominal mass of 120 from compounds with nominal masses of 121 or 119. In the case described above however, these techniques alone would not be able to distinguish the two analytes.
You might also like…
This becomes important when considering the wider bioanalytical landscape. Developments in analytical equipment have led to the introduction of coupled techniques. With the latest liquid and gas chromatography technologies allowing for the coupling of time resolved separations to mass spectrometry, the additional selectivity granted by HRMS can be critical.
Does the existence of high-resolution mass spectrometry preclude the use of ‘conventional’ mass spectrometry?
Compared to conventional mass spectrometry, HRMS offers additional information that can facilitate the confirmation of targeted species, identify unknowns and narrow down possible chemical formulas. As described in Simone Esposito’s (IRBM SpA, Pomezia, Italy) editorial, this extra information can be critical in applications where a lack of fragmentation or insufficient selectivity is observed when using MRM systems [2].
In the editorial, Esposito goes on to outline the use of HRMS in the DMPK studies of novel peptide-based modalities. Applications such as these are likely to see increased use as the drug landscape continues to evolve and more ‘undruggable targets’ are discovered.
Another potential application for HRMS is in routine untargeted analysis. As Bertand Rochat describes in his editorial, in the early era of HRMS, many other MS instruments were far superior for routine determinations and other quantitative analyses [3]. The effect of which was to mainly limit the use of HRMS to accurate mass determination, structure elucidation and other discovery-related research.
Rochat’s editorial expertly summarizes the latest developments in HRMS and puts forward a strong argument for its use in routine analysis. You can read the full editorial here.
With unrivalled selectivity and technological breakthroughs bringing routine analysis by HRMS to the fore, what does the future hold for conventional mass spectrometry techniques?
Despite the capabilities of HRMS, conventional mass spectrometry is far from obsolete. Take, for example the bioanalytical workhorse, triple quadrupole mass spectrometry (QqQ). As discussed, HRMS provides a number of benefits, yet there remain plenty of situations where the use of QqQ can be preferential.
Based on sensitivity alone, QqQ has the upper hand. Furthermore, as a ‘work horse’ instrument, assays are generally transferred to the QqQ.
For a full comparison of QqQ and HRMS, take a look at our In the Zone feature exploring this topic. This feature evaluates the merits of both types of mass spectrometry for small and large molecule bioanalysis.
You might also like…
How does high-resolution mass spectrometry work?
As a mass spectrometry technique, HRMS operates on the same basic principles as conventional mass spectrometry. In reality different mass spectrometers operate in different ways, however, all mass spectrometers broadly involve the same processes. These processes can be broken down into four stages, including ionization, acceleration, deflection and detection.
Ionization
Under a vacuum, the sample being analyzing is placed into the mass spectrometer. There are a wide variety of ionization techniques, including those that generate negative ions.
Typically, the sample is subjected to a beam of electrons. As the electrons from the beam collide with atoms in the sample, some electrons from these atoms are knocked out, generating ions.
It is important that ionization takes place under a vacuum so that the ions can travel freely without interacting with particles in the air.
Acceleration
Once the ions have been generated, they enter an electric field, which accelerates the ions. The amount the ions are accelerated depends on their charge. Highly charged ions interact more strongly with the electric field and are therefore accelerated the most. The result is that ions are separated according to their charge.
In a typical mass spectrometer, most ions have a +1 charge, since removing another electron from a positively charged ion requires more energy. Ions with +2 and +3 etc charges are possible but do not make up the majority of species in the spectrometer.
Deflection
Following acceleration in the electric field, the ions pass into a magnetic field. When the moving ions enter the magnetic field, they are deflected according to their mass and their charge. Lighter ions and ions which are more positively charged are deflected more strongly.
Detection
Finally, the beam of ions passing through the machine is detected by a computerized, electrical detector. By varying the magnetic field, it is possible to detect each ion stream as a current proportional to the number of ions arriving.
Since deflection depends on the mass of the ion and the charge on the ion, these two parameters combine to give the mass/charge ratio (m/z). The output from a mass spectrometer is generally given as a diagram showing relative intensity against m/z.
For more HRMS content, make sure you don’t miss…
1 Yang L, Amad M, Winnik WM et al. Investigation of an enhanced resolution triple quadrupole mass spectrometer for high-throughput liquid chromatography/tandem mass spectrometry assays. Rapid. Commun. Mass Spectrom. 16(21), 2060–2066 (2002).
2. https://www.bioanalysis-zone.com/2019/10/23/dmpk-hrms-essential-tool_spotl_hrms_dmpk/
3 https://www.bioanalysis-zone.com/2019/10/14/untargeted-analyses_spotl_hrms_dmpk/
Our expert opinion collection provides you with in-depth articles written by authors from across the field of bioanalysis. Our expert opinions are perfect for those wanting a comprehensive, written review of a topic or looking for perspective pieces from our regular contributors.
See an article that catches your eye? Read any of our articles below for free.