Examining the dominant bioanalytical methods for Oligo development
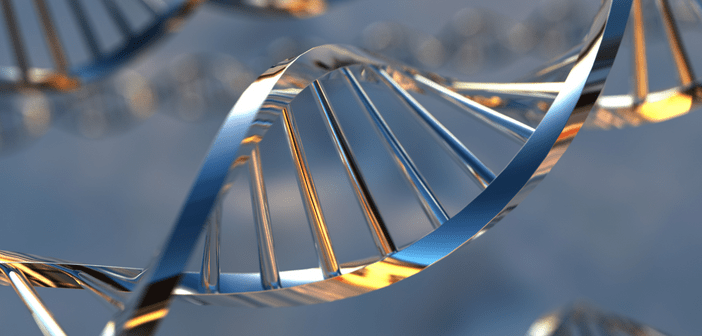
Oligonucleotides are short strands of synthetic DNA or RNA that target nucleic acids and proteins to inhibit gene expression, regulate mRNA protein functions, and manage a wide range of diseases. Specifically, “oligos” target cancer, metabolic disorders, genetic diseases and otherwise “undruggable” conditions.
It is easy to see why excitement around oligos has been building recently, but these therapeutic options have been around since the 1960s. In 1998, the U.S. FDA approved “Fomivirsen” as the first oligo-based therapeutic for cytomegalovirus retinitis. Fomivirsen has since been removed from the market due to questions about safety. Still, after scientists improved oligos’ delivery systems and stability challenges, these therapies saw a resurgence in popularity in the 2010s. As of April 2021, The U.S. FDA has approved 13 oligo therapies for various medical indications, and 223 clinical programs are underway.
Oligos are diverse and effective therapeutic solutions whose uses are constantly evolving. Scientists must adopt a straightforward, purposeful approach when designing bioanalytical strategies for oligos because the molecular target, sensitivity requirement and biological matrix all contribute to what constitutes the best approach for a specific drug.
Additional challenges to consider
Oligos’ mechanism of action relies mainly on Watson-Crick base pairing to targeted mRNA molecules. This pairing causes gene silencing, a steric block, or altered splicing patterns. However, aptamers function differently—they fold into three-dimensional shapes, engulf disease-causing mRNA and inhibit protein interactions.
Oligonucleotide therapeutics are negatively charged, hydrophilic, and can be broken into four categories that range in molecular weight.
- Antisense oligonucleotides (ASOs): 4k-10kDa
- Small interference RNAs (siRNA): ~14kDa
- Aptamers: 5k-15kDa
- Micro RNAs (miRNA): 14K-18kDa
ASO SiRNA miRNA Aptamers
Unlike many traditional small- and large-molecule therapeutics, oligos remain relatively unstable. Thus, improving stability towards degradation is one of the most critical challenges scientists face—especially in the early development phases.
Various approaches are applied and optimized to improve oligos’ circulation to improve stability. For example, oligos contain an “oligoanionic backbone” that inhibits their ability to penetrate cellular membranes. This makes it difficult for them to achieve their therapeutic goals. But chemical modifications can be made to a DNA’s phosphate-sugar backbone to minimize nuclease-mediated degradation. And to overcome excretion from oligos’ negative charges, scientists can introduce positive charges into the nucleic acids, enabling them to mask anionic motifs and achieve zwitterionic nucleic acid analogs.
Drug delivery is another crucial consideration when developing oligos. Most oligo therapies only work on nucleic acids, meaning they must enter the cell, distribute within it, and reach effective concentration at the cognate site of the RNA target. As a result, effective delivery is critical, often presenting significant challenges for preclinical development. These challenges also offer tremendous opportunities to refine and optimize delivery methods. For example, many oligos conjugate with lipid nanoparticles (LNPs), or the stealthier polyethylene glycol (PEGs), to evade the immune system, improve circulation and maximize cellular penetration. But some of these delivery systems can add toxicity, which needs to be detected and analyzed as part of a study protocol.
Better understanding immunogenicity is another critical step in successfully developing oligos. Natural immunologic responses can create anti-drug antibodies (ADA), impacting the product’s pharmacologic and toxicologic effects. The biological and chemical structures of oligonucleotides render them less immunogenic than conventional protein therapeutic products. But, there isn’t a universally accepted immunogenicity assay for oligonucleotides.
Designing a bioanalytical approach for oligonucleotides
Oligos are diverse, and their mechanisms of action are broad. They can differ in length from 15 to more than 1000 nucleotides, and they conjugate with various delivery systems to find their targets and perform their tasks. They also impact biological matrices and metabolites in significantly different ways. For all these reasons, there isn’t a single bioanalytical approach for every biologic in the oligo class. A combination of techniques will be most effective to design a thoughtful bioanalytical strategy that considers the stability, delivery and immunogenicity concerns mentioned above. The assays and tools available to scientists each have advantages and disadvantages.
Mass spectrometry (MS)-based methods
The primary advantage of MS-based bioanalysis is that it is very mass accurate and specific. Mass spectrometry can clearly distinguish between oligo intact mass and other interference, i.e., N-1 truncated metabolite, and provide data on impurities and degradation that may be invisible to ELISA and PCR methods. Another advantage of MS-based methods is that the platform is multiplex. It can quantify several analytes in one run, i.e., antisense strand, sense strand, and metabolites in the case of siRNA. The method is usually applicable for oligos whose length is less than 30 mer units. However, the methods are limited to specific instrumentation and the scientists trained to use them. These methods also use a 96-well plate during analysis, reducing throughput compared to ELISA and PCR methods. The most used MS-based methods include:
- LC-high resolution MS: Very high mass resolution. Great tool for metabolite identification during early testing stages but not as sensitive as triple quadruple (QqQ) -based LC-MS/MS method.
- MALDI-TOF-MS: Very easy to handle and provides fast analysis and turnaround times. It is not as sensitive as the QqQ-based LC-MS/MS method as well.
- LC-MS/MS: One of the most sensitive MS-based assays up to around 5 nanograms per milliliter. It is usually used in conjunction with ion-pairing chromatographic separation whose method development is very tricky. The type and concentration of ion-paring reagent need to be carefully chosen and optimized.
Hybridization immunoassays
These ligand-binding assays provide high sensitivity at around 50 pg/ml but can struggle with selectivity. They use locked nucleic acid (LNA) enhancements to target small or highly similar RNA or DNA molecules (or fragments), yet they cannot detect truncated drug metabolites. Hybridization-based assays can analyze large oligos (20-40 mer units) and they tolerate chemical modifications well. They can also produce results faster and with greater frequency than qPCR assays. The most common hybridization-based assays include:
- Ligation-free hybridization assay: Usually suitable for ASOs longer than 25 mer.
- Washable hybridization assay: Usually suitable for shorter oligos. The detection probe has low affinity with capture probe and can be washed away unless it is properly ligated to the drug.
- Nuclease-based hybridization assay: Usually applied for the analysis of an ASO shorter than 25 mer. It requires a T4 ligase to connect the drug and detection probe, and use S1 nuclease to differentiate the oligo metabolites from full-length analytes.
Hybridization fluorescence detector (FLD) methods
A PNA probe with fluorophore is used to hybrid with oligo drugs, thus enabling the hybridization product to be detected by the fluorescence detector. It offers better sensitivity (around 0.1-0.5 ng/ml) than MS-based method while keeping the advantage of the multiplex feature. It can detect large, full-length oligos (20-40 mer units) as well as truncated metabolites. Fluorescent probes are easy to use and the FLD method is much more reproducible compared to the ion-paring LC-MS/MS method. But it takes significantly longer chromatographic time to separate the metabolites than other methods, impacting its throughput. It is a good option when one needs to balance the sensitivity and specificity of the bioanalytical method.
Quantitative polymerase chain reaction (qPCR) assays
qPCR assays also use fluorescent detection to analyze and quantify oligos and biomarkers in a wide range of biological matrices. The assays measure the intensity of the fluorescence emitted and how quickly it builds up during each cycle.
These assays are highly sensitive and precise—able to detect ~0.1 picograms per milliliter—but they cannot measure truncated metabolites. And they can be inefficient when DNA/RNA fragments are shorter than 18 base pairs (bp). Scientists can use stem-loop structures to prolong oligo length and attempt a reverse PCR assay if necessary.
Utilizing instruments containing 384-well plates, throughput for qPCR assays is unparalleled. While sensitivity is the primary advantage of these assays, it is rare for drug developers to require such low levels of detection. Often, hybrid ELISA or hybrid mass spectrometry methods provide the appropriate sensitivity. qPCR assays are usually used to evaluate mRNA levels—the target regulated by oligo drugs—for pharmacodynamics (PD) purposes. Finally, regulatory guidance on validating qPCR assays has been limited, so drug developers and sponsors need to stay apprised of any emerging standards.
Areas where further research is needed
Given oligos’ unique structures, functions and modes of action, several areas require further research. This article aims not to answer these outstanding questions but to provide fodder for further discussion.
First, classifying oligos as small or large molecules is complex and confusing. The process to develop oligos is similar to that used to make small molecules, but their in vivo interaction is more closely related to large molecules. The pharmacological activity, stability, and permeability of small molecules depend on chemistry more than pharmacokinetic interactions. Small molecules also enjoy high bioavailability and can be administered orally. Yet large molecules’ pharmacodynamic specificity offers a considerable advantage in treating exactly what ails a patient. Moreover, the chief motivation for developing many large molecule drugs is to treat diseases previously thought to be untreatable. At this point, oligos should be categorized as both large and small molecules, and their use is customized to the study goal and condition they are designed to treat.
Second, we cannot regulate oligos if we cannot define them. Bioanalytical analyses of oligos often draw upon multiple methods or techniques that will undoubtedly generate unique data sets. While regulators do not expect identical data from every oligo bioanalysis, they will likely expect comparable data.
For example, mass spectrometry assays designed to detect full oligos and metabolites should consistently generate that data regardless of other techniques. Likewise, oligos requiring NLPs, PEGs or viral vectors as part of their delivery systems should invariably include PK studies to account for the added toxicity those systems may generate. The lack of regulatory guidance from the U.S. FDA or ICH has driven most developers to rely upon small molecule regulatory guidance. After all, potency, efficacy, in vivo stability and PK/PD side effects must be ascertained. But scientists, academics, regulators and drug developers must continue to drive this discussion in the name of safety and reproducibility.
Third, ASOs have two distinct mechanisms of action. ASOs fight diseases by degrading mRNA and by inhibiting mRNA via a steric block. This duplicity is because ASOs enter cells through Watson-Crick-based pairing to protein receptors but also by being released into cell cytoplasm through fluid sacks or cysts called vesicles. This phenomenon allows ASOs to achieve the highest target specificity and target previously inaccessible diseases. A better understanding of how ASOs, unlike any other oligo, can be deployed in two ways would advance oligo research greatly.
A final word on oligos
Because there is no dominant bioanalytical strategy for researching oligos, every strategy used should be developed with clinical and preclinical considerations in mind. Preclinical activities are designed to identify lead candidates, optimize formulations, develop manufacturing protocols, and determine dose routes, frequency, exposure and duration. Just like creating other therapeutics, the data from these preclinical studies help initiate the critical steps scientists take in clinical settings.
Even considering their low immunogenicity, delivery challenges and stability hurdles, oligonucleotide research should be pursued with thoughtfulness and vigor. The promise they bring to treat undruggable diseases is too great to abandon. Drug developers and sponsors with no experience or capacity to conduct the necessary studies dynamically and according to Good Laboratory Practices (GLP) should contact a proven laboratory testing partner to help.
In association with: