Comparison of selectivity and efficiency: two key factors in determining chromatographic resolution of components of a mixture
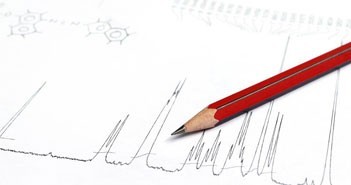
Jim Taylor is a senior chromatography specialist in the Bioanalysis and Metabolite ID group for . (UK). He has over 20 years experience in the field of liquid chromatography, comprising of 15 years LC–MS bioanalytical experience; with 11 of those years gained within a regulated contract research environment. After doing an MSc in on-line pre-concentration of pesticides, he then gained his PhD in extraction of nucleic acids using silica-magnetite nanocomposites. As an expert in the field of LC–MS bioanalysis, his primary interests include LC stationary-phase chemistries and retention mechanisms, and the influence of LC mobile-phase composition on API ionisation efficiency. He is a member of The Royal Society of Chemistry (UK), The Chromatographic Society (UK) and the British Mass Spectrometry Society.
Several years ago I was attending a meeting organized on behalf of a well-known manufacturer of mixed-mode HPLC columns. During one of the presentations, the company director explained the reason for not producing their stationary phases in smaller particle sizes was because they can achieve good separation of components of a mixture through their wide range of versatile column chemistries, rather than having to reduce particle size to achieve resolution. In technical terms; increasing the selectivity factor of the resolution equation, rather than the efficiency factor.
This made me ponder the merits of this approach and why different manufacturers appear to mainly focus on expanding their range through either: altering the physical characteristics (reduced particle size, core-shell, etc) of current stationary-phase chemistries (e.g., making them more efficient), or by expanding their range of available stationary phases with new and novel surface chemistries (e.g., creating different selectivities).
In this article I shall endeavor to appraise these two key factors that are so critical in resolving components of a mixture and how to tap into them effectively to help simplify and improve chromatographic separations.
The first thing to consider is how these factors affect separation. This is illustrated using the general resolution equation, Equation 1:
Rs = (√N/4)(α-1/α)(k/k+1)
Equation 1 – where Rs is the resolution, N is the number of theoretical plates a column possess, α is selectivity, which describes the separation of two species (A and B) on the column and k is the retention factor, a measure of the retention of a specific peak relative to an unretained peak.
This equation can be viewed as three distinct terms (or factors); the first being the ‘efficiency’, the second being the ‘selectivity’ and the third being the ‘retention’. The retention factor, k, has arguably the least impact on resolution, and its significance is reduced as compounds become more retained. In fact, at retention factors of k > 10 the impact on changing this term on resolution becomes almost negligible. So that brings us back to the other two factors in the resolution equation; efficiency and selectivity.
Efficiency, N, is given as the number of theoretical plates a column has, which in turn determines its resolving power. It is calculated using the following equation, Equation 2:
N = 16(tR/wb)2
Equation 2 – where tR is the retention time and wb is the peak width at its base.
In order to see where the ‘efficiency’ term in the general resolution equation (Equation 1) derives from, one only has to rearrange the efficiency equation (Equation 2) to solve for, (tR/wb). Thus the ‘efficiency’ term in the general resolution equation can be viewed as simply the ratio of retention time to peak width at its base. This substituted term can be isolated and adapted to give another familiar resolution equation, Equation 3:
Rs = (tR2– tR1)/(wb2+ wb1)/2
Equation 3 is simply the difference in retention time between two peaks of interest divided by the average peak width of these two peaks.
Ok, after a bit of mathematical digression, what does this actually mean for increasing efficiency to improve resolution? Well, first the bad news: resolution is not directly proportional to efficiency. Based on the general resolution equation (Equation 1) this means that every time the efficiency (N) is doubled, the resolution only increases by a factor of 1.41. Ironically, efficiency is not a very efficient way of obtaining resolution! The good news is that it’s relatively easy to increase the column efficiency while utilizing the same column chemistry/mobile phase combination. Efficiency is related to the ratio of column length to particle diameter. Therefore, doubling column length or halving particle diameter will double its efficiency.
One reason smaller particles have become so popular is that resolution can be increased without resorting to longer columns and increased run times. For example, a 50 mm column with 2.5 µm particles will give the same efficiency as a 100 mm column with 5 µm particles but with roughly half the run time (or twice the efficiency in the same run time). The use of smaller particles also has the added advantage of producing sharper peaks with less tailing/band broadening and therefore increased S/N (signal intensity/noise variation at baseline).
The rise of superficially porous particles (SPP) – also known as core-shell or pellicular – is a result of their increased efficiency for a given column length and particle diameter over fully-porous particles. This is due to their ability to attain lower plate heights and has been attributed to their superior packing ability, narrower particle size distribution, and improved mass transfer kinetics compared to fully porous particles. Touching on the subject of plate heights, it seems appropriate to briefly mention the all too familiar van Deemter equation. This relates plate height to various physical (kinetic) and chemical (thermodynamic) mechanisms that can affect the journey of a molecule through the LC column as, Equation 4:
H = A + B/u + Cu
Equation 4 – where H is plate height, A is Eddy diffusion, B is longitudinal diffusion, C is mass transfer resistance and u is mobile phase linear velocity for a particular column.
Plate height is directly related to efficiency (N) by dividing column length by plate height. Furthermore, the van Deemter equation (Equation 4) also tells us that efficiency can be increased by utilizing the optimum flow rate or linear velocity (u) for a particular column. This can be governed by many factors such as: particle diameter, porosity, size distribution and packing efficiency, as well as analyte properties such as its diffusion coefficient.
Finally, and arguably the most versatile – if not most interesting – way to increase resolution is by altering the selectivity of a chromatographic method. Selectivity (α) is simply defined as the retention factor (k) ratio of two peaks of interest. The ability to alter the selectivity of a method is the most powerful tool in any chromatographer’s toolbox. There are many ways to achieve this; however, the factors which have greatest influence and effect are: stationary-phase chemistry, organic modifier, and aqueous phase pH/ionic strength. Unfortunately many chromatographers do not use these factors to their advantage often enough.
These days there are a multitude of different stationary-phase chemistries involving various perturbations around the bonded-phase and base material combinations (underestimate the selectivity effects of the base material – silica, polymeric, hybrid, silica-hydride, zirconia, etc., – at your own peril!). The stationary-phase has a profound effect on retention and selectivity ranging over all modes including: Reversed-phase (C18/C8, PFP, Polar-embedded, Phenyl), Ion-exchange/mixed-mode (IEX-RP, IEX-NP), HILIC/Normal-phase (unbonded silica, ion-exchange), and ANP (PFP, silica-hydride). Stationary-phase surface chemistry allows the chromatographer to tap into certain secondary interactions, such as π-π/aromatic (e.g. Phenyl, PFP), hydrogen-bonding (e.g. Polar-embedded), steric/shape selectivity (e.g. PFP), and ion-exchange/electrostatic (e.g. mixed-mode, PFP) – not to mention the additional Lewis acid/base interactions gained from using a zirconia base instead of the more commonly used silica base.
Of course half the battle is knowing how to bring the best out of your column with the correct use of mobile phase. Acetonitrile will compete with and disrupt any selectivity gains from π–π interactions when using a phenyl or PFP column to resolve an aromatic mixture (such as structural isomers). Whereas methanol will promote these interactions allowing for resolution of aromatic structural isomers. However, acetonitrile will help promote HILIC interactions over methanol to an extent that high acetonitrile concentrations can cause HILIC interactions with residual silanols on a reversed-phase column. In addition, acetonitrile will allow for different degrees of interaction with a polar-embedded phase – or any polar or ionic functionality of the support – compared with using methanol. Of course other organic modifiers, such as acetone or IPA, will also impart their own effect on selectivity.
The use of aqueous-phase modifiers to influence factors such as pH and ionic strength can also be extremely powerful in orchestrating a separation. Careful manipulation of pH to promote or subdue ionisable groups, so as to alter one compound’s retention over the other compound, can cause two co-eluting peaks to spring apart as if they were like-poles of a magnet. Likewise the careful control of ionic strength can allow the chromatographer to tweak the extent to which ion-exchange interactions from the column come into play.
It should be noted that related parameters such as temperature and buffer counter-ion can also have profound effects on selectivity (especially, for example, in chiral separations, or by influencing the analyte form/structure). The key prerequisite, however, to using selectivity to your full advantage is in knowing the physical and chemical characteristics of your compounds of interest. Stumbling around the chromatographic minefield without knowing each compound’s various functionalities, their pKa, and the compound’s LogD profile, can turn out to be a very lengthy, frustrating experience!
In conclusion, is one approach better than the other; efficiency vs selectivity? The answer in my mind is no. Both have their part to play in any chromatographic system. Efficiency may not be the most ’efficient‘, but its gains are easy enough to achieve. Even good LC practice of minimizing extra-column volumes will help improve resolution of any method. Selectivity on the other hand is a far more powerful resource to dig into and certainly comes into its own when well-retained co-eluting compounds are showing no signs of resolving. It does however require some knowledge of the sorbent/solute/solvent chemistry triangle and how to take advantage of various parameters to implement with full effect. However, armed with a bag of tricks and a good measure of background knowledge, any separation should be a walk in the park!