Capillary electrophoresis-mass spectrometry in metabolomics: highlighting the sweet spots
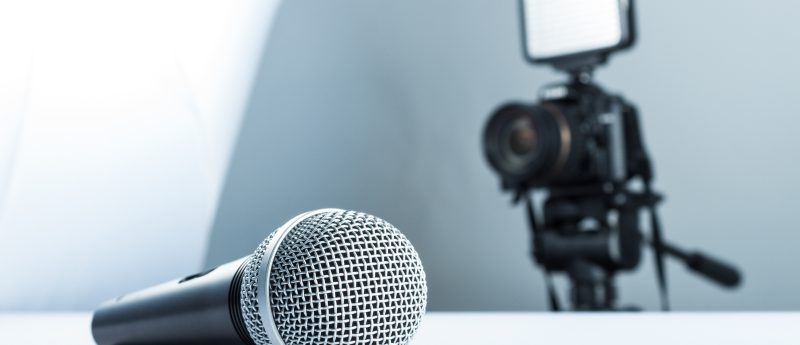
Rawi Ramautar
Biomedical Microscale Analytics
Leiden Academic Centre for Drug Research
Leiden University, The NetherlandsRawi Ramautar obtained his PhD on the development of capillary electrophoresis-mass spectrometry methods for metabolomics from Utrecht University, the Netherlands, in 2010. In 2013 and 2017, he received the prestigious Veni and Vidi research grants from the Netherlands Organization for Scientific Research for the development of CE-MS approaches for volume-restricted metabolomics. Currently, he is a principal investigator at the Leiden University (The Netherlands) where his group is developing microscale analytical workflows for sample-restricted biomedical problems. Rawi Ramautar was recently selected for the Top 40 under 40 Power List of the Analytical Scientist.
Keywords: capillary electrophoresis; chiral metabolome; mass spectrometry; polar metabolome; volume-restricted samples
Probing the polar metabolome
The efficient and selective analysis of charged and highly polar metabolites remains a challenge with the standard analytical toolbox employed in metabolomics [1,2]. Capillary zone electrophoresis (CE), a microscale analytical technique, separates compounds according to their intrinsic electrophoretic mobility, which, in essence, is dependent on the compound size and charge. Therefore, CE has the right analytical characteristics for the profiling of polar ionogenic metabolites under biocompatible conditions. In this context, CE-MS is considered an orthogonal analytical separation method, i.e. when used in conjunction with the more established chromatographic-based approaches, additional or complementary metabolomics information will be provided for a given sample, notably for the polar metabolome [3,4]. For example, Drouin et al. recently compared the metabolic coverage of CE-MS to HILIC-MS and reversed-phase LC-MS by analyzing a standard metabolite mixture composed of 596 compounds with diverse physico-chemical properties. In this study, it revealed that CE-MS performed well for the analysis of polar ionogenic metabolites, including amino acids, nucleotides, sugar phosphates and sulfated compounds [4]. Nucleotides and sugar phosphates are still very difficult to analyze with the standard analytical techniques and, therefore, CE-MS can play a key role here in metabolomics.
With the advent of improved interfacing designs for coupling MS and CE over the last decade [5], CE-MS-based workflows have been developed for in-depth analysis of polar ionogenic metabolites in a wide range of biological samples [6–8]. For example, Zhang et al. demonstrated that CE-MS employing a sheathless porous tip interface enabled the highly sensitive analysis of nucleotides, including compounds like ATP, ADP and AMP. Detection of the analytes was performed in the positive ion mode, which circumvented corona discharge, a phenomenon that is often observed in nano-ESI-MS under low-flow separation conditions and with detection in negative ion mode [9]. By using an injection volume of only 6.5nl, sub-nanomolar detection limits for nucleotides could be obtained with plate numbers above 200,000 thereby clearly illustrating the high separation efficiency of CE.
Addressing biomass-restricted metabolomics studies
Metabolomics studies often encounter difficulties due to the limited sample material or volume available for analysis. For example, microfluidic 3D-cell culture models utilizing patient-derived cells are increasingly used to address biological questions. These microfluidic cell culture systems often deal with low numbers of (mammalian) cells, which are typically around a few hundreds to thousands of cells. Understanding the behavior of a small population of cells or (ultimately) of a single cell within a tumor is another important example where advanced microscale analytics is urgently needed in order to gain insight into the biological process with metabolomics.
CE-MS is a powerful microscale analytical tool for addressing material and/or volume-restricted biomedical and clinical problems. In general, nanoliter injection volumes are used from just a few microliters of material when utilizing micro/nanovials, making CE-MS well-suited for profiling metabolites in tiny sample amounts. Low nanomolar detection sensitivities for a wide range of polar metabolite classes can be easily obtained with recently developed CE-MS methods, as shown for applications dealing with low number of mammalian cells to even a single cell, despite the low injection volumes [6–8,10].
Another application area where CE-MS can be of high value is neuroscience, where transgenic mouse models for various neurological diseases are often employed [11]. Body fluids like microdialysates and cerebrospinal fluid are of great value here in order to gain insight into the pathological mechanism underlying brain disorders. Clearly, these body fluids can only be obtained in limited amounts, often less than 1µl. Also for these kinds of volume-restricted samples, CE-MS has revealed its potential for in-depth metabolic profiling studies, as demonstrated for mouse cerebrospinal fluid (CSF) [12]. In this case, the collected mouse CSF was only diluted with water (1:1, v/v), thereby keeping the sample intact, and by using an injection volume of about 45nl over 300 compounds can be detected. By only consuming such a small volume for injection, the CE-MS approach permitted multiple injections from the same sample, enabling the recording of technical replicates but also to profile the same sample at different CE-MS separation conditions, allowing the extraction of more biochemical information. The ability to perform multiple analyses with CE-MS on an often precious volume-restricted sample is not possible with the standard analytical toolbox used in metabolomics.
Metabolomics studies concerned with low amounts of biological sample need to carefully address pre-analytical steps, notably for checking loss of analytes due to potential adsorption effects and also issues related to volume mismatch, i.e. the sample is often further diluted during the pre-analytical steps. In the latter case it would be important to use an injection strategy that allows the relevant part of the biomass-restricted sample to be efficiently injected into the CE-MS system. As such, miniaturization and optimization of sample preparation will be of pivotal importance for biomass-restricted metabolomics, in addition to the use of electrokinetic injections. Overall, I anticipate that the use of pre-analytics fully adapted to volume-restricted samples with recently developed CE-MS approaches will enable the reliable analysis of metabolites in low numbers of mammalian cells (to even a single cell). Moreover, such methodological advances will be of high value to single cell biopsies and nanodosing studies, notably in cancer research.
Sample too salty? CE-MS can do the job
An elegant characteristic of CE-MS is the ability to selectively preconcentrate acidic or basic metabolites in the CE capillary using techniques like transient-isotachophoresis and dynamic pH-junction [13,14]. These preconcentration steps can yield at least a tenfold improvement in sensitivity and are fully compatible with salt-rich samples. For example, van Mever et al. recently developed a CE-MS method for the analysis of endogenous metabolites in rat brain microdialysates without using any pre-analytics [15]. In order to improve the detection sensitivity, a dynamic pH-junction preconcentration step was considered and optimized. Theprocedure was fully compatible with the high-salt concentration present in rat brain microdialysate samples. Low nanomolar detection limits were obtained for amino acids when an injection volume of 291nl was used, which corresponded to 17% of the total capillary volume, without compromising the high separation efficiency of CE (i.e. plate numbers above 300,000 for the amino acids). The CE-MS approach enabled the detection of 48 compounds in a rat brain microdialysate sample of which 25 could be tentatively identified and quantified.
Looking into the mirror of the metabolome
In metabolomics, the focus has been primarily on the analysis of the L-Metabolome (apart from various sugars). However, gradually more and more studies are indicating that chiral endogenous metabolites, especially D-amino acids, are playing an important role in modulating mammalian physiology and pathology. CE-MS has been used for the selective profiling of D- and L-amino acids in various biological samples using chiral selectors or chiral derivatization reagents [16–18]. In general, in comparison to L-amino acids, D-amino acids are present at trace levels in biological samples. Therefore, highly sensitive and selective CE-MS approaches are needed for this purpose. CE-MS systems using a sheathless porous tip nanospray emitter have shown their value for in-depth metabolic profiling of volume-limited biological samples [19]. It is therefore anticipated that these CE-MS methods will play a key role in determining the presence and functions of D-amino acids in biological systems.
Acknowledgements
Rawi Ramautar would like to acknowledge the financial support of the Vidi grant scheme of the Netherlands Organization for Scientific Research (NWO Vidi 723.016.003).
The opinions expressed in this feature are those of the author and do not necessarily reflect the views of Bioanalysis Zone or Future Science Group.
References
- Hirayama A, Wakayama M and Soga T. Metabolome analysis based on capillary electrophoresis-mass spectrometry. Trends Analyt. Chem. 61, 215–222 (2014).
- Kuehnbaum NL and Britz-McKibbin P. New advances in separation science for metabolomics: resolving chemical diversity in a post-genomic era. Chem. Rev. 113(4), 2437–2468 (2013).
- Ramautar R, Nevedomskaya E, Mayboroda OA et al. Metabolic profiling of human urine by CE-MS using a positively charged capillary coating and comparison with UPLC-MS. Mol. Biosyst. 7(1), 194–199 (2011).
- Drouin N, Pezzatti J, Gagnebin Y, Gonzalez-Ruiz V, Schappler J and Rudaz S. Effective mobility as a robust criterion for compound annotation and identification in metabolomics: toward a mobility-based library. Anal. Chim. Acta, 1032, 178–187 (2018).
- Lindenburg PW, Haselberg R, Rozing G and Ramautar R. Developments in interfacing designs for CE-MS: towards enabling tools for proteomics and metabolomics. Chromatographia, 78, 367–377 (2015).
- Onjiko RM, Portero EP, Moody SA and Nemes P. In situ microprobe single-cell capillary electrophoresis mass spectrometry: metabolic reorganization in single differentiating cells in the live vertebrate (Xenopus laevis) embryo. Anal. Chem. 89(13), 7069–7076 (2017).
- Zhang W, Guled F, Hankemeier T and Ramautar R. Utility of sheathless capillary electrophoresis-mass spectrometry for metabolic profiling of limited sample amounts. J. Chromatogr. B Analyt. Technol. Biomed. Life Sci. 1105, 10–14 (2019).
- Kawai T, Ota N, Okada K et al. Ultrasensitive single cell metabolomics by capillary electrophoresis-mass spectrometry with a thin-walled tapered emitter and large-volume dual sample preconcentration. Anal. Chem. 91(16), 10564–10572 (2019).
- Zhang W, Guled F, Hankemeier T and Ramautar R. Profiling nucleotides in low numbers of mammalian cells by sheathless CE-MS in positive ion mode: circumventing corona discharge. Electrophoresis, 41(5–6), 360–369 (2020).
- Liu JX, Aerts JT, Rubakhin SS, Zhang XX and Sweedler JV. Analysis of endogenous nucleotides by single cell capillary electrophoresis-mass spectrometry. Analyst, 139(22), 5835–5842 (2014).
- Romanova EV, Sweedler JV. Animal model systems in neuroscience. ACS Chem. Neurosci. 9(8), 1869–1870 (2018).
- Ramautar R, Shyti R, Schoenmaker B et al. Metabolic profiling of mouse cerebrospinal fluid by sheathless CE-MS. Anal. Bioanal. Chem. 404(10), 2895–2900 (2012).
- Ramautar R, Busnel JM, Deelder AM and Mayboroda OA. Enhancing the coverage of the urinary metabolome by sheathless capillary electrophoresis-mass spectrometry. Anal. Chem. 84(2), 885–892 (2012).
- Baidoo EE, Benke PI, Neususs C et al. Capillary electrophoresis-fourier transform ion cyclotron resonance mass spectrometry for the identification of cationic metabolites via a pH-mediated stacking-transient isotachophoretic method. Anal. Chem. 80(9), 3112–3122 (2008).
- van Mever M, Segers K, Drouin N et al. Direct profiling of endogenous metabolites in rat brain microdialysis samples by capillary electrophoresis-mass spectrometry with on-line preconcentration. Microchemical Journal, 156, 104949 (2020).
- Castro-Puyana M and Marina ML. Chiral capillary electrophoresis-mass spectrometry. Methods Mol. Biol. 1985, 391–405 (2019).
- Sanchez-Hernandez L, Serra NS, Marina ML and Crego AL. Enantiomeric separation of free L- and D-amino acids in hydrolyzed protein fertilizers by capillary electrophoresis tandem mass spectrometry. J. Agric. Food Chem. 61(21), 5022–5030 (2013).
- Prior A, Moldovan RC, Crommen J et al. Enantioselective capillary electrophoresis-mass spectrometry of amino acids in cerebrospinal fluid using a chiral derivatizing agent and volatile surfactant. Anal. Chim. Acta, 940, 150–158 (2016).
- Ramautar R. Resolving volume-restricted metabolomics using sheathless capillary electrophoresis-mass spectrometry. LC GC Eur. 30(12), 658–661 (2017).
Our expert opinion collection provides you with in-depth articles written by authors from across the field of bioanalysis. Our expert opinions are perfect for those wanting a comprehensive, written review of a topic or looking for perspective pieces from our regular contributors.
See an article that catches your eye? Read any of our articles below for free.