Paper-based assays as useful tools for clinical bioanalysis
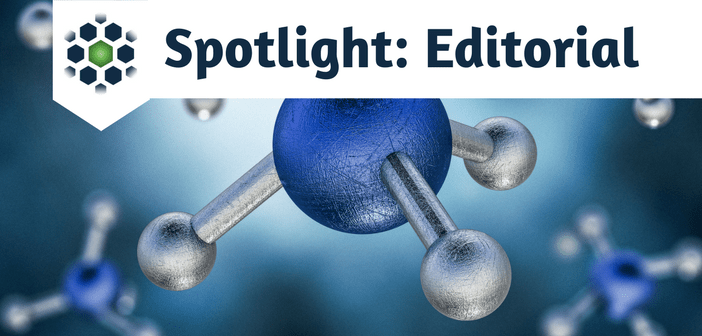
Dr Adaris M. López Marzo was a student at Nanobioelectronic & Biosensors Group and received her PhD in Chemistry from the Autonomous University of Barcelona (Spain, 2013). She earned a Master Degree in Analytical Chemistry (2004) and a Bachelor in Radiochemistry (1999) from the University of Havana and the Higher Institute of Nuclear’s Science and Technology (Cuba). She is interested in developing novel nanostructured materials and POC biosensors devices for environmental and biomedical applications. She worked as postdoctoral researcher at the Institute of Advanced Chemistry of Catalonia (IQAC)-Spanish National Research Council (CSIC) and is now working as postdoctoral research at Vall d´Hebron Institut de Reserca (Barcelona, Spain). She is an author of 16 indexed scientific articles (h=8, SCJ) some of them related to the development of biosensors and nanomaterials-based bioconjugates for biosensor applications.
The development of adequate diagnostic tools for the early detection of disease biomarkers and the adequate follow-up of their treatments is one of the major challenges of global health care. Many efforts in health-care research are focused towards the development of personalized medicine and person centered care, two notions that, in recent decades, have expressed ambitions to tailor the design of patient care, and align the strategies of diagnosis and treatment to the individual needs of each patient [1,2]. To meet these ambitions, there is an enormous demand for new, highly sensitive, portable, economical and reproducible biosensors. Point-of-care (POC) analytical devices for real-time monitoring of illness biomarkers, which can be operated by any individual in any place, are highly desired [3]. These are very small analytical tools of easy portability and low cost, which are simple to operate by unskilled individuals with little training, without the necessity of additional reagents or analytical instruments to give an accurate analytical result.
In this context, paper-based sensors have gained popularity as an effective alternative to POC. The low cost and simplicity of their fabrication together with the benefits of the final product justify the great interest invested in these devices. The analysis speed, as well as the flexibility and versatility of formats have made them attractive and easy-to-use analytical tools, with possibilities for miniaturization and mass-production as portable screening methods for POC testing [4-6].
At their early stage of development, paper-based assays were mostly used to give qualitative or semi-quantitative yes/no (i.e. positive/negative) results based fundamentally on colorimetric response with low limits of detection (LoD). However, the sensitivity of this technology has increased with the introduction of other methods of detection such as electrochemistry, electroconductivity, fluorescence, photoluminescence, chemiluminescence and electrochemiluminescence amongst others [4,7,8], in addition to the incorporation of nanomaterials (such as gold nanoparticles, silver nanoparticles, quantum dots) as carriers of detection elements or signal producers [3-5].
Paper-based assays can be sorted into three groups in accordance with their analytical format:
- Dipstick: The first paper-based assay was based on the impregnation of reagents on paper and the change in the color signal when the analyte was applied (glucose (Glc) test, 1957). In 1982, immunorecognition was first introduced to dipsticks by immobilizing antibodies on a paper membrane, which increased the specificity and range of detectable analytes [7].
- Lateral Flow Assays (LFAs): Capillary-driven lateral flow (LF) was introduced to conventional dipstick technology in 1989. Here, the capillary action is the main motion force for the movement of liquids within the paper pores. LF eliminated the incubation and washing steps of the standard protocols of dipstick-based assays. It also increased the total number of captured and detected analyte molecules in a given time, improving the LoDs [3,7].
- Microfluidic paper-based analytical devices (µPADs): In 2007, μPADs were introduced by G. Whitesides and co/workers at Harvard University (MA, USA). They are made by forming hydrophobic points, channels or areas in hydrophilic paper using photolithography, plotting, plasma etching, inkjet printing, cutting, wax printing and other techniques [3,4,8]. In these devices a large variety of innovative and fantastic architectures overcoming the disadvantages of the initial and classical formats (dipstick and LF), has been developed maintaining the simplicity of the operation and the low cost of fabrication. In µPADs the integration with other disciplines has made the development of many multifunctional devices possible with high-performance analysis including very low LoD, very small sample volumes, the possibility of multicomponent analyses and the automatization of sequential-multistep procedures generating all-integrated devices.
The real-life applications of paper-based assays are unquestionable. Actually, many assays and reader accessories are successfully commercialized by various companies, used both in health care centers and by patients at home:
- Multi-test urinary dipsticks involving different reagent-deposited zones are commercialized for Glc, albumin, pH, leukocytes, nitrite, ketones, urobilinogen, bilirubin, specific gravity and blood. For example, URiSCAN Strips (for 12 urine-analytes) [9], Chemstrip® (for Glc) [10], Multistix® 10SG Reagent Strips and CLINITEK Microalbumin 9 Reagent Strips that involve ten and nine, respectively, urine-tests for managing patients with diabetes and kidney disease [11].
- Products like ACCU-CHEK Performa system [10], FreeStyle brand [12] and Glucostix® [13] have been commercialized for glc testing in blood by Roche Diagnostics, Abott and Bayer Diabetes-Care, respectively.
- AccuBio Tech Co., Ltd. sells rapid LFAs for detecting approximately 15 infectious diseases, over 20 drugs of abuse in urine and saliva, PSA and CEA cancer biomarkers, cardiac markers (cTnI, CK-MB and Myoglobin) and analytes related with the fertility (hCG pregnancy, Luteinizing hormone of ovulation, Follicle-stimulating hormone and Toxo IgG) [14]. Preventis GmbH commercializes LFIAs for cTnI, high-sensitivityC-reactive protein (hsCRP) and D-dimer (all of them useful for diagnosis of cardiovascular diseases). In addition, tests for detecting thyroid stimulating hormones, the specific antibody against mutated citrullinated vimentin (anti-MCV) used for the diagnosis of rheumatoid arthritis, the Helicobacter. pylori antigen for intestinal disorders, Chlamydia infection and many other assays are commercialized too [15].
- Digital readers (e. g. Bio-AMD DSR from Bio-Alternative Medical Devices) [16] and smartphone accessories (e. g. HRDR-200 from Cellmic) [17] for LFA devices are commercialized too.
Although dipstick and LF formats have dominated the rapid diagnostics market over the last three decades (as exemplified with the real-life applications listed above), recently, some examples of commercial μPADs applications have emerged as versatile POC platforms. Some companies have taken advantage of the variety of academic studies based on lab-on-a-chip and μPADs with attractive advances over the classical formats. Fortunately, many of these interesting academic studies have been introduced into the market by companies currently developing the next generation of technologies. For example, Alere, Abaxis, Abbott, Achira labs, Agilent, Biosite, Chembio and Daktari Diagnostics have produced devices via the integration of microfluidic and lab-on-a-chip technologies with paper-based assays. Diagnostics for All is an example of a non-profit company creating and commercializing POC medical-diagnostic devices. Devices for routine monitoring of liver function (controlling HSA, transaminases and lactate dehydrogenase), devices for the isolation, amplification, and detection of DNA and RNA sequences for the detection of infectious disease, devices for controlling micronutrients (such as iron, zinc, iodine and vitamin A) and devices for the successful vaccination against tetanus and measles are produced and commercialized by Diagnostics for All.
Despite the several μPADs advantages, vast amount of academic research focused on them and the increasing number of studies conducted, the industrial transfer of some of these devices is still a challenge. The entry of μPADs into the market is still very limited due to multiple factors defining commercialization strategies. Some researchers believe that the discrepancy between the highly-active academic developments presented by the μPADs and their entry into real-life applications lies on aspects of operational simplicity, long-term stability of devices and that academic research often ends up in proof-of-concept demonstrations without practical analysis [18].
In my opinion, there are actually many devices developed in the academic lab, protected by patents, ready to be the subject of technology transfer studies. However, the industries require a mature market in a particular clinical area, to assess and then shift from the current diagnostic methods to the subsequent implementation of the new ones. The change in the existing production lines for a company always represents high costs (infrastructure, proprietary technology, etc.) despite significant technological advantages that could be represented by the use of paper. I believe that the real-life introduction of µPADs, like the most versatile trend of paper-based assays, will escalate and become more common. It is important to remember that this technology is quite young, (just 10 years old) with respect to dipstick and LF, which are between 50 and 60 years older. It is still in its infancy and the average patients are still not aware of the few products currently available on the market. In this context, the integration of µPADs with smartphones will help to disseminate these devices globally. Smartphones offer important advantages to µPADs including high resolution cameras, multiple modes of wireless communication, excellent data storage capacity, processing and graphics power to support application software and many hours of battery. They could be utilized for automated sample logging and data processing, as well as the storage and shipment of results for subsequent interpretation by highly-skilled personnel (doctors, nurses), both locally and internationally. The assembly of smartphones with µPADs will help to meet the expectation of demand of POC biosensors for clinical bioanalysis in the field of personalized medicine.
Acknowledgements: Adaris M. López Marzo thanks the Carlos III Institute for awarding her the Sara Borrell postdoctoral fellowship.
References:
[1] El-Alti L, Sandman L, Munthe C. Person Centered Care and Personalized Medicine: Irreconcilable Opposites or Potential Companions? Health Care Anal., DOI 10.1007/s10728-017-0347-5 (2017) [Epub ahead of print]
[2] Aziz MA, Yousef Z, Saleh AM, Mohammad S, Al Knawy B. Towards personalized medicine of colorectal cancer. Crit. Rev. Oncol. Hematol. 118:70-78 (2017)
[3] Hu J, Wang S, Wang L, et al. Advances in paper-based point-of-care diagnostics. Biosens. Bioelectron. 54:585-597 (2014)
[4] López-Marzo AM and Merkoçi A. Paper-based sensors and assays: a success of the engineering design and the convergence of knowledge areas. Lab Chip. 16:3150-3176 (2016)
[5] Liang P, Yu H, Guntupalli B, Xiao Y. Paper-Based Device for Rapid Visualization of NADH Based on Dissolution of Gold Nanoparticles. ACS Appl. Mater. Interfaces. 7:15023-15030 (2015)
[6] Yang Y, Noviana E, Nguyen MP, Geiss BJ, Dandy DS, Henry CS. Paper-Based Microfluidic Devices: Emerging Themes and Applications. Anal. Chem. 89: 71-91 (2017)
[7] Yetisen AK, Akram MS, Lowe CR. Paper-based microfluidic point-of-care diagnostic devices. Lab Chip., 13: 2210-2251 (2013)
[8] Martinez AW, Phillips ST, Whitesides GM, Carrilho E. Diagnostics for the developing world: microfluidic paper-based analytical devices. Anal. Chem. 82:3-10 (2010)
[9] YD Diagnostics: www.yd-diagnostics.com/2012/eng/ (Accessed 9 November 2017)
[10] https://usdiagnostics.roche.com/en/point_of_care_testing.html#Urinalysis
[11] www.healthcare.siemens.com/point-of-care/urinalysis
[12] www.abbott.com/our-products/consumers/freestyle.html
[13] http://diabetes.bayer.com.ar/productos/glucostix.php
[14] www.accubiotech.com/templates/T_yestem_News/index.aspx?nodeid=39
[15] www.preventis-online.de/en/prevention-check/prevention/overview/
[16] Bio-AMD, DSR: www.bioamd.com/technology
[17] Cellmic, HRDR-200: www.cellmic.com/content/rapidtest-solution/hrdr-200-chromatographic-immunoassay-reader/
[18] Yamada K, Shibata H, Suzuki K, Citterio D. Toward practical application of paper-based microfluidics for medical diagnostics: state-of-the-art and challenges. Lab Chip. 17(7):1206-1210 (2017)
Our expert opinion collection provides you with in-depth articles written by authors from across the field of bioanalysis. Our expert opinions are perfect for those wanting a comprehensive, written review of a topic or looking for perspective pieces from our regular contributors.
See an article that catches your eye? Read any of our articles below for free.