Analyte identification strategies that complement imaging mass spectrometry
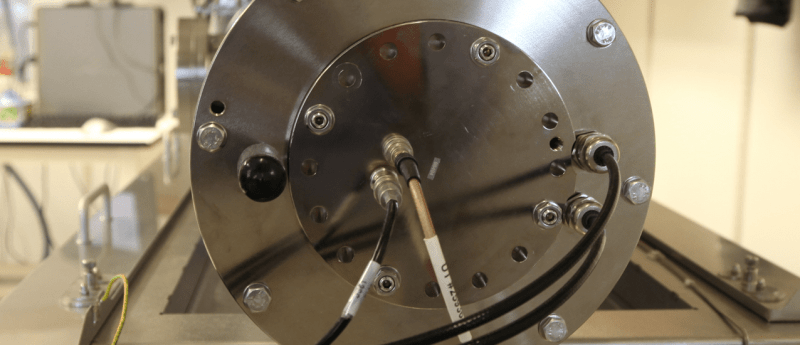
In this latest instalment of our column from the Mass Spectrometry Research Center at Vanderbilt University, Danielle Gutierrez discusses several analyte identification strategies and how they complement imaging mass spectrometry.
The complexity of biological systems presents a formidable challenge to understand the matrices of life. The application of mass spectrometry to help answer biological questions facilitates the acquisition of detailed molecular information, for example, the identification of specific protein isoforms or metabolites and the localization of distinct sites of post-translational modifications. In addition to this information, imaging mass spectrometry (IMS) reveals the distribution of these molecular species within cells or tissues.
Since its early days, a challenge within the field of IMS has been to identify the analytes imaged from tissue sections. While a parallel LC–MS/MS experiment on a tissue sample homogenate is usually feasible, this approach does not preserve the spatial localization at the resolution of the image. In addition, this can dilute signal of the desired analyte or can make it difficult to unequivocally equate an identification with an imaged analyte. To overcome these limitations, several strategies discussed below identify analytes directly from tissue sections.
Hydrogel technology
Recently, Harris et al., developed a strategy to identify analytes directly from tissue sections using hydrogel technology coupled with mass spectrometry [1]. In this approach, proteins are digested and extracted directly from tissue sections using small (~1–4 mm in diameter) trypsin loaded hydrogel disks [1–3]. Typically, the hydrogels are placed on a tissue section (after mounting it to a slide or MALDI target) to digest and extract analytes in the covered region. Digestion using hydrogels is feasible by conventional methods, for example storing the tissue section with the placed hydrogel in a humidity chamber at 37–50°C for 4 h to overnight [1–3], and by a 2 minute microwave digestion [3]. After digestion, peptides are extracted from the gel and can be analyzed using LC–MS/MS [1,2] or MALDI MS [1,3].
The hydrogel approach can be used in parallel with IMS by carrying out digestion and extraction on an adjacent tissue section, or subsequent to imaging on the same tissue section [1]. Hydrogels can provide an in-depth analysis of a tissue region of interest that is not easily obtained by IMS alone. For example, an on-tissue hydrogel extraction and digestion followed by LC-MS/MS analysis identified peptides from over 100 proteins with a molecular weight of >50 kDa, a quarter of which had molecular weights >100 kDa and many of which were membrane proteins [1]. Continuing work with hydrogel fabrication seeks to decrease the diameter of the disks to permit their placement on tissue sections at higher spatial resolution [1].
Liquid microextraction
An additional approach to identify proteins directly from tissue regions of interest is liquid microextraction. For this procedure, a small droplet of solvent is placed onto a tissue section while maintaining contact with a gel-loading tip. The solvent remains on the tissue for a brief period to extract analytes, which are then diluted and analyzed by LC–MS/MS [4]. Using this technique, proteins from 1–2µL extractions of lens, brain, and kidney tissues were identified by top-down LC–MS/MS. In this case, the extraction area was approximately 2 mm in diameter and picomoles of protein were extracted [4]. This strategy can also be applied after on-tissue digestion for a bottom-up approach.
Liquid extraction surface analysis (LESA) represents a commercialized version of this strategy. The technique is conducted in an automated fashion using a robotic sampling device, the TriVersa Nanomate by Advion, to directly pipette solvent onto and aspirate it off of the tissue surface [5]. The size of the extraction droplet depends on the type and volume of solvent used and the height from which the solvent is placed. Examples from the literature report LESA droplet sizes ranging from 0.4 mm to <4 mm in diameter [6–8].
Direct imaging MS/MS
Analyte identification can also be obtained by MS/MS analysis during the imaging experiment. One example using an ion trap mass spectrometer is the identification of promethazine from mouse brain tissue at 100 µm spatial resolution [9]. Here, promethazine was isolated with a targeted MS scan and subsequently dissociated to obtain characteristic fragment ions for accurate identification. The resulting images of promethazine distribution across brain sections displayed the summed intensity of two promethazine fragment ions [9].
Ion trap mass spectrometers are particularly well-suited for small molecule analysis as they can perform MSn, which may be required in some cases to differentiate various small molecules of the same or similar m/z ratio. In another example, a TOF/TOF mass spectrometer was used to image and identify the small molecule drug rifampicin and a galactosyl sulfatide [10]. This work demonstrates the advantages of using MS/MS within an imaging experiment, namely an increase in molecular specificity and the potential to reduce the background noise observed in MS mode by acquiring signals in MS/MS mode [10].
Identification guided by high mass accuracy
High mass accuracy is of advantage for identification whether a strategy is spatially-directed (as described above) or comprehensive (e.g., performed on an entire tissue sample or tissue section). Recent examples demonstrate the use of high mass accuracy for the identification of proteins observed in imaging experiments. Using an indirect identification approach, rat brain and mouse kidney tissues were either imaged in the high mass range (m/z 1,000-15,000) via MALDI FTICR IMS or homogenized and analyzed by top-down LC–MS/MS [11]. In these cases, distinct localization and identification of several modified proteins were achieved, including localization of an oxidized form of S100A8 to Staphylococcus aureus microcolonies in the mouse kidney [11].
The utility of high-mass accuracy for identification was also demonstrated with on-tissue digestion IMS and bottom-up LC-MS/MS analysis [12]. Furthermore a recently created database, the MSiMass List [13], can assist in the identification of protein signals using the high mass accuracy of analytes from imaging experiments without additional identification information [14].
Conclusion
Several strategies for identification of imaged analytes are presented here, and a recent review discusses the importance of high mass accuracy as well as additional approaches to facilitate identification of imaged analytes (e.g., use of ion mobility mass spectrometry to separate isobaric peptides and laser capture microdissection) [15].
The decision to pursue a particular identification strategy should be based on the biological complexity of the samples and the questions to be answered, the requirements set forth at the start of the experiment, and the available resources. Over the last five years, progress on the identification of analytes directly from tissue has advanced greatly and continued development of direct-tissue analysis techniques and imaging instrumentation will greatly improve these capabilities.
References
- Harris GA, Nicklay JJ, Caprioli RM. A localized in-situ hydrogel-mediated protein digestion and extraction technique for on-tissue analysis. Anal. Chem. 85(5), 2717–2723 (2013).
- Nicklay JJ, Harris GA, Schey KL, Caprioli RM. MALDI imaging and in situ identification of integral membrane proteins from rat brain tissue sections. Anal. Chem. 85(15), 7191–7196 (2013).
- Taverna D, Norris JL, Caprioli RM. Histology-directed microwave assisted enzymatic protein digestion for MALDI MS analysis of mammalian tissue. Anal. Chem. 87(1), 670–676 (2015).
- Schey KL, Anderson DM, Rose KL. Spatially-directed protein identification from tissue sections by top-down LC-MS/MS with electron transfer dissociation. Anal. Chem. 85(14), 6767–6774 (2013).
- Advion. Mode 3: Liquid Extraction Surface Analysis (LESA®). Available from: <https://advion.com/products/triversa-nanomate/mode-3-liquid-extraction-surface-analysis-lesa/>.
- Swales JG, Tucker JW, Spreadborough MJ, et al. Mapping drug distribution in brain tissue using liquid extraction surface analysis mass spectrometry imaging. Anal. Chem. 87(19), 10146–10152 (2015).
- Randall EC, Bunch J, Cooper HJ. Direct analysis of intact proteins from Escherichia coli colonies by liquid extraction surface analysis mass spectrometry. Anal. Chem. 86(21), 10504–10510 (2014).
- Griffiths RL, Cooper HJ. Direct tissue profiling of protein complexes: Toward native mass spectrometry imaging. Anal. Chem. 88(1), 606–609 (2016).
- McClure RA, Chumbley CW, Reyzer ML, et al. Identification of promethazine as an amyloid-binding molecule using a fluorescence high-throughput assay and MALDI imaging mass spectrometry. NeuroImage Clin. 2, 620–629 (2013).
- Prentice BM, Chumbley CW, Caprioli RM. High-speed MALDI MS/MS imaging mass spectrometry using continuous raster sampling. J. Mass Spectrom. 50(4), 703–710 (2015).
- Spraggins JM, Rizzo DG, Moore JL, et al. MALDI FTICR IMS of intact proteins: Using mass accuracy to link protein images with proteomics data. J. Am. Soc. Mass Spectrom. 26(6), 974–985 (2015).
- Schober Y, Schramm T, Spengler B, Römpp A. Protein identification by accurate mass matrix-assisted laser desorption/ionization imaging of tryptic peptides. Rapid Commun. Mass Spectrom. 25(17), 2475–2483 (2011).
- McDonnell LA, Walch A, Stoeckli M, Corthals GL. MSiMass list: a public database of identifications for protein MALDI MS imaging. J. Proteome Res. 13(2), 1138–1142 (2014).
- Spraggins JM, Rizzo DG, Moore JL, Noto MJ, Skaar EP, Caprioli RM. Next-generation technologies for spatial proteomics: Integrating ultra-high speed MALDI-TOF and high mass resolution MALDI FTICR imaging mass spectrometry for protein analysis. Proteomics 16, 1678–1689 (2016).
- Mascini NE, Heeren RMA. Protein identification in mass-spectrometry imaging. TrAC Trends Anal. Chem. 40, 28–37 (2012).